INTRODUCTION
Polyploidy has played an important role in plant evolution and its study has relevance in diverse fields, such as cytogenetics, physiology, plant reproduction, cytotaxonomy, biogeography and breeding of cultivated plants (Ramsey & Schemske, 2002, 2014; Soltis et al., 2014; McAllister et al., 2015; Chansler et al., 2016). The enormous plant biodiversity has occurred due to genomic duplications that happened in the evolutionary past (Alix et al., 2017). Polyploidy has usually been considered as a dead end in plant evolution (Soltis et al., 2014). Nevertheless, if polyploids become established, reaching reproductive maturity and producing a small percentage of fertile pollen grains, they can reproduce and leave polyploid offspring, or cross with their diploid parents that gave rise to them and increase the polyploid proportion within the original population (Madlung & Wendel, 2013). Speciation through this pathway is best known as polyploid evolution (Soltis & Soltis, 1995; Ramsey & Schemske, 1998; Ainouche & Wendel, 2014; Lavania, 2020) and is a very common phenomenon in the family Asteraceae (Pellicer et al., 2010; Mas de Xaxars et al., 2016; Barker et al., 2016; Huang et al., 2016). The tribe Vernonieae (Asteraceae) is one of the groups of the South American taxa with about 25% of polyploids (Jones, 1979b). Within this tribe, Lessingianthus H. Rob. is the genus with the greatest proportion of polyploids (nearly 60%) known so far (Angulo & Dematteis, 2012). Lessingianthus comprises more than 133 species, which are perennial herbs, shrubs or erect subshrubs that live mainly in tropical South America, with a high concentration of species in eastern Brazil (Robinson, 1988).
Chromosome counts reported for the genus Lessingianthus showed a great variation in the ploidy levels of the species, ranging from diploid (2n = 2x = 32) to 1-decaploid (2n = 11x = 176), and they are all based on the basic chromosome number x = 16 (Angulo & Dematteis, 2012; Pérez et al., 2021). Many species of the genus have high ploidy levels (6x, 8x, 10x) and a taxon with an odd chromosome complement and high ploidy level has also been reported (2n =11x) (Dematteis, 1996, 1997, 2002, 2009; Dematteis & Fernández, 2000; Dematteis et al., 2007; Angulo, 2012; Angulo & Dematteis, 2009a, 2009b, 2010, 2012, 2013, 2015). In addition to the large variation in the chromosome number observed, Lessingianthus also shows intraspecific chromosome variation and has species with different ploidy levels, such as L. sellowii (Less.) H. Rob. and L. rubricaulis (Bonpl.) H. Rob., with reported diploid and tetraploid cytotypes (Angulo & Dematteis, 2012). In order to understand the evolution of a polyploid species it is essential to know their origin (Jauhar & Joppa, 1996). The analyses of the effects of polyploidy on the fertility of the species are scarce, as well as the documented relevance of polyploidy in the genus.
Polyploid evolution is considered a major force of speciation in natural populations (Lavania, 2020). This type of speciation is clear in Lessingianthus if we consider the number of polyploids with high ploidy levels. Despite the large amount of cytological information available for both Lessingianthus and the tribe that confirms the usefulness of chromosomal characters in taxonomic and evolutionary studies (Jones, 1979; Turner et al., 1979; Galiano & Hunziker, 1987; Ruas et al., 1991; Dematteis, 1998, 2002; Dematteis & Fernández, 2000; Dematteis et al., 2007; Oliveira et al., 2007a, 2007b; Angulo & Dematteis, 2009a, 2009b, 2012, 2015; Vía do Pico & Dematteis, 2012, 2013, 2014), there is little information that contributes to unraveling the mechanisms of origin and establishment of these natural polyploid populations. Establishment refers to the mechanisms underlying the spread or colonization of new populations by neopolyploids (Kreiner et al., 2017). Several authors have used meiotic behavior to clarify these mechanisms in other plant groups (Pagliarini, 2000; Ramsey, 2007; Ortiz et al., 2011; Kovalsky & Solís Neffa, 2012; Via Do Pico & Dematteis, 2012; Farco & Dematteis, 2014). Previous studies on meiotic behavior included a few entities of the genus (Angulo, 2012; Pérez et al., 2021). Data available on the meiotic behavior of these natural polyploids is scarce and fragmented. The studies related to the meiotic behavior of Lessingianthus might help to understand the complex chromosomal evolutionary pathway and the mechanisms of formation and establishment of associated polyploid populations.
Floral morphological traits are commonly used in the taxonomy of the tribe Vernonieae, which are related to the inflorescence, involucral bracts, receptacle, corolla, stamen and the achene (Keeley & Robinson, 2009). Angulo & Dematteis (2014) analyzed the variability of floral microcharacters in the genus Lessingianthus with taxonomic purposes and concluded that the shape of the apical appendage, in combination with other characters, has diagnostic value that differentiates between morphologically similar species. Considering the scarce published literature on comparative anatomy and floral morphology of the tribe Vernonieae (Misra, 1972; Martins & Oliveira, 2007; Galastri & Oliveira, 2010), we noticed that the reproductive biology of this morphologically diverse group had not yet been studied in depth. Understanding the embryological development of the species can help us to determine the effect of the polyploidy phenomenon on pollen fertility: Is there an abnormal development or behavior?, and if so, is it exclusively due to events during meiosis of the pollen mother cells, or alterations in the development of the anthers?. These are interesting questions from an embryological point of view, however embryological studies are scarce and most of the entities analyzed are from the Old World (Palm, 1925; Tiagi & Taimni, 1960, 1963; Pullahiah, 1979). To date, the only study on reproductive characters of the natural tetraploid L. plantaginodes (Less.) H. Rob. has helped to determine its polyploid nature due to the consistent results that agree with a diplodized autopolyploid specimen (Pérez et al., 2021). Considering the paucity of information, the study of the male reproductive characters of a larger number of species is necessary to understand the taxonomic implications and to provide highlights on the reproductive traits of species with different ploidy levels of the genus.
In view of the scarce cytoembryological knowledge of the genus we perform a developmental study of anthers with the main objective of study if the different ploidy level affect the anther and pollen development in Lessingianthus species; this information will provide future insights into the role of polyploidy in the evolution of this genus. Finally, we infer the male fertility of diploid and polyploid Lessingianthus species. This study not only contributes to the characterization of the sporangium and male gametophyte development, but also enhances the knowledge of the reproductive biology of the genus with taxonomic purpose.
MATERIALS AND METHODS
Plant material
The flowers of the material studied are reunited in heads surrounded by numerous phyllaries. Each flower is perfect, tubular, isomorphic, sessile and epigynous. The androecium of all the analyzed species is formed of five introrse and connate anthers; each anther is bithecal and tetrasporangiate, has an oblong shape and presents longitudinal dehiscence. They are attached to each other forming a staminal tube around the style, a condition called synanthereous androecium.
Ten species of diploid (2x) and polyploid (4x, 6x, 8x and 10x) taxa of the genus of Lessingianthus from different localities in Argentina and Paraguay were analyzed. Information about the studied material, voucher specimens and reference of the previous chromosome counts is provided in Table 1. Vouchers are deposited in the herbarium of the Instituto de Botánica del Nordeste (CTES; Thiers, 2022).
For the meiotic studies, ten flower buds per species were fixed in 1:5 lactic acid-ethanol (Fernández, 1973) and stored in 70% ethanol at 4°C. For the anatomical analyses, 20-30 heads with floral buds and open flowers were fixed in FAA (70% alcohol, formalin, acetic acid, 90:5:5).
Anatomical analysis
The material was dehydrated in an ascending series of alcohols and embedded in paraffin (Johansen, 1940; modified by González & Cristóbal, 1997). Serial transverse sections of 10-12 μm thick were cut with a rotary microtome. The sections were stained with Safranin-Astra blue (Luque et al., 1996) and then mounted with synthetic Canada balsam. The slides were examined under a Leica DM LB2 (Leica, Wetzlar, Germany) light microscope equipped with a digital camera. We used the terminology proposed by Maheshwary (1950) and Pullaiah (1979a) to describe the male gametophyte development.

Table 1 Chromosome count, ploidy level, locality details, and voucher information of Lessingianthus species analyzed in this study. Chromosome numbers of analyzed species are based on the references cited in the table. Abbreviations: (a) meiotic analysis; (b): tetrad analysis; (c): pollen stainability; (d): gametophyte development. *References: description of chromosome number.
Meiotic and sporad analysis
The meiotic behavior was analyzed at meiosis I and II of the Lessingianthus studied species; simultaneously, the microspore mother cell (MMC) with regular and irregular behaviors were counted.
Anthers with MMCs were squashed and stained with 2% lactopropionic orcein (Dyer, 1963). Slides were examined and photographed using a Zeiss Axioplan microscope (Carl Zeiss, Jena, Germany) with a Canon Power Shot A 640 digital camera (Tokyo, Japan). Analyses included meiotic behavior at different stages and tetrad formation. We considered those with four equal-sized microspores as normal tetrads.
RESULTS
In the following, we will first describe the development of the anther, sporogenesis and gametogenesis, and finally the results of the analysis of male fertility.
Anther development
The development of the gametophyte and anther wall was observed in different species of Lessingianthus (Table 1). All the species analyzed presented the same development pattern, both in the anther wall and in the formation of pollen grains.
The anther primordia are composed of a group of undifferentiated, isodiametric cells, with thin walls and dense cytoplasm (Fig. 1A). Each staminal primordium is comprised of three layers that developed in the different tissues of the mature anther: the outer layer (L1), the subepidermal layer (L2) and the innermost layer (L3) (Fig. 1A). The first layer L1 originated the protodermis through mitotic anticlinal divisions (Fig. 1B). During the development, this layer gave rise to epidermis by successive anticlinal divisions (Fig. 1C). At the same time, the mitotic divisions in the subepidermal layer L2 and L3 increased in the cells of the central region of the primordium, thus giving the characteristic tetralobed appearance of anthers (Fig. 1B, C). Mitotic divisions in each lobule of L2 gave rise to a row of archesporial cells (Fig. 1B, C). These cells were voluminous, with dense cytoplasm and prominent nuclei (Fig. 1B, C, D). The innermost layer L3 differentiated the procambium, forming a central vascular bundle (Fig. 1B-E). This layer also gave rise to the connective tissue and septum located between the microsporangia.
Microsporangia wall development
The row of archesporial cells underwent mitotic divisions and gave rise to two cell lines: the internal primary sporogenous cell and the external primary parietal cell (Fig. 1E, F). The first increased in size and directly became sporogenous tissue that consisted of MMC (Fig. 1G, H, I). The latter suffered periclinal divisions and originated two secondary parietal layers: the internal secondary parietal layer oriented through the central region of the anther, and the external secondary parietal layer located towards the periphery of the anther (Fig. 1G).
In the young tetralobed anther the internal secondary parietal layer differentiated into tapetum (Fig. 1H, I). Meanwhile, the external secondary parietal layer is divided into two layers (Fig. 1G, H): the external layer that originated the endothecium and the internal layer that gave rise to the middle layer (Fig. 1G, H, I).
In this way, the anther wall consisted of four layers: epidermis, endothecium, middle layer and tapetum (Figs. 1H, I and 2A).
Epidermis: consists of a layer covered by a thin cuticle, with rectangular cells of dense cytoplasm and conspicuous nuclei (Fig. 2A). At the dehiscence stage, this layer was observed obliterated above the endothecium (Fig. 2G).
Endothecium: this layer originated from external secondary parietal cell division as well as the middle layer (Fig. 2G, H). This layer consisted of rectangular elongated cells with prominent nuclei (Fig.2 A). At the two-celled pollen grain stage the endothecium cells increased in size and lignified thickening was deposited on the walls (Fig. 2F).

Fig. 1 Anther wall development of Lessingianthus species. A-C, G-I: L. rubricaulis. D-F: L. teyucuarensis. A, anther primordia showing L1, L2 ang L3 layers. B, staminal primordium with protodermis and archesporial cell of subepidermal location. C, tetralobed anther with archesporial cell, epidermis and procambium. D, anther with a voluminous archesporial cell. E, anther showing division of the archesporial cell. F, primary sporogenous cell and primary parietal cell. G, microsporangia with internal and external secondary parietal layers; the asterisk showed the division of the external parietal cell. H, microsporangia cell wall with four differentiated cell layers: epidermis, endothecium, middle layer and tapetum; the arrow showed the division of the external parietal cell. I, mature anther wall observed in the longitudinal section. Abbreviations: ar, archesporial cell; ep, epidermis; en, endothecium; esp, external secondary parietal layer; isp, internal secondary parietal layer; L1, outer layer; L2, subepidermal layer; L3, innermost layer 3; MMC, microspore mother cell; ml, middle layer; pd, protodermis; pr, procambium; ppc, primary parietal cell; psc, primary sporogenous cell; spt, sporogenous tissue; ta, tapetum; *, external secondary parietal layer in division. Scales: A = 20 μm; B-I = 50 μm. Color version at http://www.ojs.darwin.edu.ar/index.php/darwiniana/article/view/1104/1286
During the anthesis stage the differential thickening of the endothecium cell walls helped to open the anther wall and release the pollen grains (Fig. 2G).
Middle layer: it was first observed at the sporogenous tissue stage (Fig. 1G, H, I). It is formed of two homogeneous layers of small cells with thin walls (Fig. 1I). Later in the development, this middle layer started to degenerate at the MMC stage (Fig. 2A); by the young tetrad stage it had collapsed and was no longer visible (Fig. 2B).
Tapetum: This layer was derived directly from the internal secondary parietal layer (Fig. 1G, H).
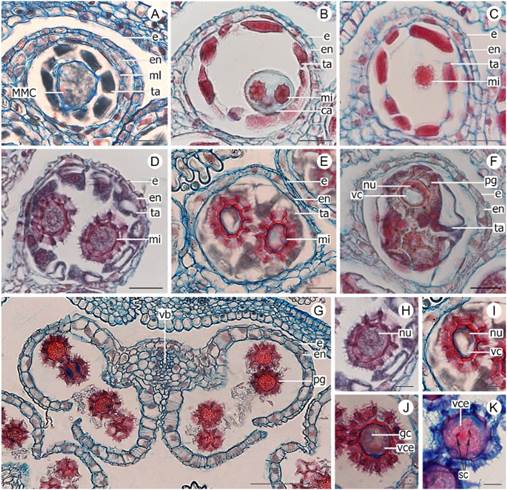
Fig. 2 Male gametophyte development of Lessingianthus species. A-C: L. niederleinii. D-F, H-J: L. teyucuarensis. G, K: L. rubricaulis. A, anther locule in cross section with MMC, showing the four layers and obliterated middle layer. B, anther locule in tetrad stage, no middle layer observed. C, a small microspore recently released from tetrad. D, anther locule with tapetum starting to envelop the one-celled microspore. E-F, anther locule completely invaded by tapetum. G, stamen in anthesis with obliterated epidermis. H, young one-celled microspore. I, microspore with prominent vacuole and nucleus displaced to the periphery. J, two-celled pollen grain with a large vegetative cell that encloses a small generative cell. K, mature pollen grain with two sperm cells and a vegetative cell. Abbreviations: ca, callose; e, epidermis; en, endothecium; gc, generative cell; mi, microspore; ml, middle layer; nu, nucleus; pg, pollen grain; MMC, microspore mother cell; sc, sperm cell; ta, tapetum; vb, vascular bundle; vc, vacuole; vce, vegetative cell. Scales: A-F = 20 μm; G = 100 μm; H-K = 10 μm. Color version at http://www.ojs.darwin.edu.ar/index.php/darwiniana/article/view/1104/1286
It consisted of a layer of rectangular cells, with dense cytoplasm and conspicuous nuclei, with high metabolic activity (Fig. 1I). At the beginning, the cells increased in size and persisted in situ (Fig. 2A); over the course of microspore stage, they started to deform and then protruded the anther locule (Fig. 2D). Afterwards, the tapetum invades the locule and completely surrounds the vacuolated microspore (Fig. 2E-F). The tapetal cells that invaded the loculus do not integrate into a true plasmodium and maintain their individuality (Fig. 2F). During the stage of two celled pollen grain, it was no longer observed (Fig. 2G).
Microsporogenesis
The MMC underwent meiotic division: meiosis I produced two reduced nuclei and meiosis II gave rise to four haploid cells. The cytokinesis was of the simultaneous type. The formed microspores were arranged in an isobilateral or tetrahedral tetrad, covered with callose (Fig. 2B). At the microspore stage the callose surrounding them dissolved and the microspores were released into the anther locule (Fig. 2C).
Microgametogenesis
The microspores had dense cytoplasm and a prominent, centrally located nucleus (Fig. 2H). The microspores grew and incorporated large amounts of water and developed a prominent vacuole that displaced the nucleus toward the periphery (Fig. 2I). The cell underwent the first mitotic division and gave rise to a large vegetative cell and a small generative cell which was enclosed inside its cytoplasm (Fig. 2J). Suddenly afterwards, a mitotic division in the generative cell gave rise to two spermatic cells (Fig. 2K). Thus, mature 3-celled pollen grains were released at anthesis (Fig. K).
Meiotic behavior
In general, meiotic behavior was highly regular in all examined species with varying percentages of meiotic regularity higher than 70% (Table 2). The phases without meiotic abnormalities were prophase I and telophase I. In the remaining phases analyzed different percentages of meiotic irregularities were observed, such as chromosomes out of plate (Fig. 3A), bridges without fragments (Fig. 3B-C), asynchrony (Fig. 3D-E) and micronucleus (Fig. 3F).

Fig. 3 Abnormal meiotic behavior of Lessingianthus species. A: L. pusillus. B, F: L. argenteus. C: L. rubricaulis. D-E: L. brevifolius. A, chromosomes out of plate in AI. B, bridges without fragments in AI. C, bridges without fragments in AII. D, asynchrony in AI. E, asynchrony in AII. F, micronucleus in TII. Abbreviations: arrowheads: chromosomes out of plate; arrow: micronucleus. Scales: A, D = 10 μm; B, C, E-F = 20 μm.
These irregularities occurred during prophase II, metaphase I and II, anaphase I and II and telophase II of the species analyzed.
In the diploid species, the irregularities were mostly concentrated in the first meiotic division. The three diploid species studied (L. brevifolius (Less.) H. Rob., L. rubricaulis and L. pusillus (Dematteis) M.B. Angulo) showed relatively low percentages of irregularities. The MMC observed at metaphase I presented only one irregularity, while anaphase I was the most unstable phase of diploid species because it presented three different meiotic irregularities (see Table 2), among which, bridges without fragment (Fig. 3B-C) was the most frequently observed. The first meiosis of L. brevifolius (2x) was highly stable while the second meiosis only showed MMC with abnormal behavior (Table 2). Lessingianthus rubricaulis (2x) presented a diversity of irregularities in both stages of meiosis and L. pusillus (2x) had the highest proportion of chromosomes out of plate (Fig. 3A; Table 2).
Polyploid specimens had different irregularities dispersed through the phases of meiosis II. The tetraploid L. pseudoincanus (Hieron.) Dematteis & M.B. Angulo presented a highly stable meiotic division with low percentages of MMCs with abnormal behavior, which mainly occurred at the second meiotic division. Among the hexaploid analyzed species, L. argenteus (Less.) H. Rob. had the most irregular behavior of all the species studied. The meiosis I of this species presented the highest percentages of abnormalities, while the meiosis II had different percentages of abnormalities (Table 2). In contrast, the other hexaploid species L. niederleinii (Hieron.) H. Rob. had high regular behavior with only two meiotic abnormalities. Finally, the species with the highest ploidy level had low abnormal meiotic behavior: octoploid L. pubescens M.B. Angulo & Dematteis, is a meiotically stable species with meiotic irregularities in low proportions and the decaploid L. teyucuarensis (Cabrera) Dematteis had no meiotic abnormalities (Table 2).
Tetrad analysis
The regular and irregular sporads formed after meiosis was counted for different Lessingianthus species. All species presented high percentages of normal tetrahedral and isobilateral tetrad formation (Fig. 4A and B, respectively; Table 3). Among the analyzed species, L. polyphyllus (Sch. Bip. ex Baker) H. Rob. (4x) and L. niederleinii (6x) presented only normal tetrads, whereas L. argenteus (6x) had the lowest tetrad formation with 49.11% (Table 3).

Fig. 4 Sporads and viable/non-viable pollen grains of Lessingianthus. A-B, E: L. polyphyllus. D: L. brevifolius. F: L. teyucuarensis. G: L. pseudoincanus. A, normal tetrahedric tetrad. B, normal isobilateral tetrad. C, abnormal triad. D, abnormal triad. E, two viable pollen grains and one empty non-viable pollen grain (arrow). F, a small and non-viable pollen grain (arrow). G, an abnormal stained pollen grain of small size (arrow). Abbreviations: arrows = abnormal pollen grains. Scales: A-B, D, F = 10 μm; C = 30 μm; E, G = 50 μm.
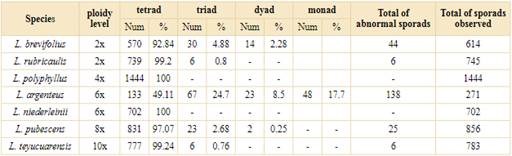
Table 3 Number and percentages of normal and abnormal sporads formation of Lessingianthus analyzed species.
Different and variable percentages of abnormal sporads were found (Table 3). All the diploids formed triads (Fig. 4C-D) and dyads. These two abnormal sporads were commonly observed in L. brevifolius, whereas L. rubricaulis only formed small percentages of triads. Polyploids formed low percentages of triads, dyads and monads (Table 3), the hexaploid L. argenteus being the only species that formed monads and which had the highest percentages of triads and dyads (Table 3).
Pollen viability
Pollen viability estimated through pollen stainability was relatively high in almost all the species studied and ranged from 78% to 99% (Table 4). The abnormal pollen grains observed were empty and unstained (Fig. 4E) or had an abnormally small size (Fig. 4F-G). The diploid species analyzed of the genus showed a viability higher than 80%. Lessingianthus bardanoides (Less.) H. Rob. was the only diploid species that presented low percentages of viable pollen grains (27.52%; Table 4). In the polyploids, the tetraploid L. pseudoincanus was the species with the highest proportion of viable pollen grains (99.15%), while the lowest proportion was observed in L. argenteus (66.45%). Pollen grains of the high ploidy level species were highly viable: L. pubescens with 98.04% and L. teyucuarensis with 78.88%.
DISCUSSION
Male gametophyte development and trends in reproductive traits
In general, the development of the male gametophyte of the species studied is uniform. The diploid and polyploid species studied displayed normal anther development.
All the species showed a dicotyledonous type of anther development. This type of development was first described by Davis (1966) and it is a common type of development for the Asteraceae family. The species of Lessingianthus studied here shares this type of development with other tribes of Asteraceae such as: Inulae, Heliantheae, Cichorieae, Lactuceae, Senecioneae and in the genus Opisthopappus C. Shih (Pullaiah, 1978, 1979a, 1979b, 1981; Yurukova-Grancharova, 2004; Yurukova-Grancharova & Dimitrova, 2006; Gotelli et al., 2008; Jiana et al., 2009; Chehregani et al., 2011; Chehregani & Hajisadeghian, 2014). However, other developmental types have also been documented in the family. The basic type was reported uniquely in Stiffia chrysantha Mikan, S. fruticosa (Vell.) D. J. N. Hind & Semir, Wunderlichia mirabilis Riedel ex Baker and W. senae Glaz. ex Maguire & G. M. Barroso (Bonifacio et al., 2018). The report of monocotyledonous type development in Ageratum conyzoides L. is even less common (Franca et al., 2015). Because of this, Bonifacio et al. (2018) considered that the basic and monocotyledonous types have evolved independently in Asteraceae, while dicotyledonous development is a plesiomorphic character due to the large number of species in the family that exhibit it.
There are two types of tapetum among Spermatophyta: glandular tapetum (also called secretory or cellular type) and invasive tapetum (also called amoeboid or true periplasmodial type) (Pacini, 2016). In the family Asteraceae, there are species with glandular tapetum (like Sonchus oleraceus L.) and different types of amoeboid tapetum (Pacini, 1997). According to Pacini (1997), the behavior of the tapetal cells of the family Asteraceae varied: there are amoeboid syncytial tapetum (as in Helianthus annuus L.), non-syncytial amoeboid tapetum (Cosmos bipinnatus Cav.) and a variant of non-syncytial amoeboid tapetum with one row of microspore mother cells (Cichorium intybus L.). The time at which the periplasmodium appears also varies between species: middle tetrad stage, early or middle microspore stage (Pacini, 1997; Furness, 2008; Wang et al., 2020). The behavior of the tapetal cells observed in Lessingianthus species studied is comparable to H. annuus tapetum, since the tapetum protrudes the anther locule at middle microspore stage after a transient parietal phase. However, we note that the cells that invade the loculus of the species studied do not integrate into a true plasmodium, since they maintain their individuality. For this reason, we consider it as a non-syncytial invasive tapetum. This type of tapetum disintegrates completely at the bicellular pollen grain stage and was reported in other species of the subtribe such as Elephantopus scaber L., Vernonia elaeagnifolia DC. and V. divergens Benth. (Pullahiah, 1979a).
In the absence of embryological data, Pérez et al. (2021) first reported male gametophyte development of L. plantaginodes. The embryological features of another species of the genus described in this study are consistent with most of the features reported by Pérez et al. (2021). Other members of the tribe Vernonieae shared these male embryological features (Pullaiah, 1979a; Pérez et al., 2021). Herr (1984) consigned a list of embryological characters of taxonomic relevance according to previous studies performed in Angiosperms (Cave 1953; Gerassimova-Navashina, 1958, 1971; Herr, 1967; Takhtajan, 1969, 1976). Based on the results of this study, we were able to determine the following features considered of taxonomic importance by Herr (1984): number of microsporangia; type of wall development; number of layers; persistence of the epidermis; formation; number and shape of tetrads. From this list, all species studied of the genus so far presented tetrasporangiate anthers, four-layered anther wall (endothecium, middle layer, epidermis and tapetum) of dicotyledonous development, with persistent epidermis, invasive tapetum, tetrahedral or isobilateral tetrads, and 3-celled pollen grains. Despite what has been observed in Lessingianthus, in the large family Asteraceae there are also bisporangiate anthers (Pullaiah, 1979b, 1983; Franca et al., 2015), monocotyledonous and basal types of anther wall development (Franca et al., 2015; Bonifacio et al., 2018), glandular tapetum (Chehregani et al., 2011; Franca et al., 2015; Bonifacio et al., 2018), decussate, isobilateral or T-shaped tetrads (Pullaiah, 1978, 1979b, 1981, 1983; Yurukova-Grancharova et al., 2006; Ao, 2007; Deng et al., 2010; Chehregani & Hajisadeghian, 2014) and mature 2-cellular pollen grains (Bonifacio et al., 2018). Although there is variability within the family related to male gametophyte development and the sporophitic structures associated, in the species studied, the main features related to reproduction are conserved. Our results confirm that the features of the male gametophyte development were uniform to all the analyzed entities. We can also affirm that polyploidy does not affect the normal development of the anthers of the species under study, since no anatomical alterations were observed during the development of the anthers.
Inferences on gamete formation: meiotic behavior and tetrads
Angulo (2012) observed few aberrations and in small proportions in hexaploid L. centauropsideus (Hieron.) M.B. Angulo & Dematteis and tetraploid L. saltensis (Hieron.) H. Rob., and other populations of L. brevifolius and L. rubricaulis. These few irregularities occurred at metaphase and anaphase in the first meiotic division; in a similar way the MMC of the diploid species studied here presented irregularities in phases of the first division. All diploid species analyzed in this work showed meiotic aberrations in the first meiosis division and this is consistent with the results reported in diploids specimens, L. brevifolius and L. rubricaulis, by Angulo (2012). In contrast, the polyploid species analyzed in this work showed a high concentration of meiotic irregularities, principally in anaphase and telophase of the second meiotic division. Only L. argenteus breaks this scheme by having meiotic irregularities at meiosis I and II. The highly irregular meiotic behavior of this species along with a low production of normal tetrads, is typical of neopolyploids (Ramsey & Schemske, 2002). Therefore, we inferred that L. argenteus might be a recently formed polyploid.
The abnormal behavior observed in this study is often associated with anaphase, the chromosomal segregation phase. Lacadena (1996) argued that this phase may be affected by the absence of homologous chromosome pairing or the anomalous behavior of chiasma (precisely the absence of crossover or precocious resolution of chiasms). This may lead to the formation of irregularities observed in our results and previously reported data of Lessingianthus, such as off-plate univalents, laggards and precocious migration of chromosomes (Angulo, 2012; Pérez et al., 2021). In relation to these findings, Darlington (1965) stated that the morphological characteristics of chromosomes may affect the correct pairing of chromosomes. In this sense, chromosome size and morphology has a significant role in the chiasma formation between homologous chromosomes: small submetacentric or telocentric chromosomes tend to form univalents (Darlington, 1965). This is in accordance with the previous karyotype analysis, which revealed the small chromosomes in the genus Lessingianthus, averaging 1.75 μm., with the submetacentric type in lower proportions (Ruas et al., 1991; Dematteis, 1996, 1997, 1998; Dematteis & Fernández, 2000; Oliveira et al., 2007; Angulo, 2012; Angulo & Dematteis, 2009a, 2009b, 2014). These two karyotype characteristics may explain in part the abnormal behavior related to the chromosome pairing and segregation.
Of all the abnormalities observed in the Lessingianthus species, chromosomes out of the equatorial plate at metaphase was one of the most frequent abnormalities. This aberration has been mentioned in the meiosis of other taxa of the family, such as Senecio L. (López et al., 2002); Cirsium Mill. (Nouroozi et al., 2011); Tithonia diversifolia (Hemsl.) A. Gray (Alcorcés de Guerra et al., 2007); Clematis montana Buch. -Ham. ex DC. (Singhal et al., 2010); Campuloclinium macrocephalum (Less) DC. (Farco & Dematteis, 2014); Cichorieae Lam. & DC. (Gupta et al., 2014); Vernonanthura twediana (Baker) H. Rob. (Vega & Dematteis, 2016); Lactuca dolicophylla Kitam, Chrysanthemum leucanthemum L. and Eupatorium adenophorum Spreng. (Gupta et al., 2017). This type of abnormality also was found in all species studied of the closely related genus Chrysolaena H. Rob. (Via Do Pico & Dematteis, 2012, 2014). The random segregation of chromosomes out of plate produces sterile aneuploid gametes, with irreversible effects on plant fertility (Ramsey & Schemske, 2002; Comai, 2005; Otto, 2007). The genetic mechanisms that overcome this instability are different, depending on the type of polyploid: auto or allopolyploid. In the former, the genetic mechanisms of the polyploid are aimed at restoring balanced chromosome segregation (2:2), whereas in allopolyploids the mechanisms tend to avoid recombination of homeologous chromosomes (Comai, 2005). In both cases, the formation of bivalents and their correct segregation is favored. Although this abnormality was observed in the species studied, it was only seen in a few MMCs, so the impact on plant fertility was considerably low. This result correlates with the high production of normal tetrads and high pollen viability found in the studied species.
Since irregularities are commonly associated with polyploids with unstable genome behavior during meiosis, the different abnormalities found in diploids studied were noteworthy (Otto & Whitton, 2000; Comai, 2005; Otto, 2007). Under such circumstances, we can state that Lessingianthus meiotic abnormalities occur independently of the ploidy level of the specimen. The proven sensitivity of meiosis to environmentally induced stress events may explain this situation, as has been suggested in several plant groups (Hoekstra, 1992; Saini, 1997; Mamun et al., 2006; Pacini & Dolferus, 2016). Different studies have shown that the climatic conditions to which the plant is exposed severely affect the different phases of meiosis (Namuco & O’Toole, 1986; Demotes-Mainard et al., 1996; Tang et al., 2011; Onyemaobi et al., 2017; Lei et al., 2020). Cold stress caused repression of dynamic factors in the cytoskeleton of wheat (Triticum aestivum L.) leading to defects in cytokinesis of meiosis I (Tang et al., 2011). In rice (Oryza sativa L.), water stress produced different abnormalities associated with a particular phase: univalents were observed in prophase I and II; lagging chromosomes were recorded in metaphase I, anaphase I and II; and formation of micronuclei in telophase I (Namuco & O’Toole, 1986). Heat stress induced unbalanced tetrad formation, polyadenylation and meiotic restitution in Arabidopsis thaliana (L.) Heynh; both chromosome segregation and cytokinesis were affected by temperatures of 36-38 °C (Lei et al., 2020). It is highly probable that heat stress is influencing meiosis in the analyzed plants, since their flowering occurs during the hottest months of the year (November to February) and the average maximum temperature at the collection sites are around 38-39 ºC, with historical records of up to 42ºC according to the national meteorological service of Argentina (https://www.smn.gob.ar/). Therefore, this phenomenon could explain the variability found in the meiotic abnormalities.
In plants, regular meiosis concludes with the formation of four haploid nuclei of similar size (John, 1990; Ohkura, 2015). After cytokinesis the four haploid cells covered with callose might have a tetrahedral, isobilateral, decussate, linear or T-shaped arrangement (Bhojwani & Bhatnagar, 1986). The species analyzed here mostly formed normal tetrads of equal size and tetrahedral or isobilateral arrangement. However, in a few cases the meiosis of diploid L. brevifolius and L. rubricaulis, hexaploid L. argenteus, octoploid L. pubescens and decaploid L. teyucuarensis produced irregular tetrads. Seijo & Solís Neffa (2006) and Ortiz et al. (2011) stated that irregular tetrads are formed as a consequence of abnormalities in previous stages. Therefore, the abnormal tetrads observed here might be linked to the meiotic abnormalities observed. The analyses performed show triads, dyads and monads in different proportions. Both diploids and polyploids formed irregular tetrads, so we can infer that the production of abnormal tetrads is independent of the ploidy level, as in the case of meiotic behavior.
The abnormal sporads scored were mainly triads. This type of sporad has two haploid cells and one diploid cell (Brownfield & Köhler, 2011). There are different possible ways to form triads: by absence of cytokinesis (Pagliarini, 2000); abnormal orientation of the axes that originate parallel or tripolar spindles at metaphase II (Kovalsky et al., 2014; Taschetto & Pagliarini, 2003); restitution nuclei induced by persistent bridges (Ortiz et al., 2011); or restitution nuclei at the second meiotic division (Kovalsky et al., 2014). The analyzed species presented bridges in the first meiotic division; however, these bridges were not observed during the second meiotic division. Additionally, no fused nuclei were observed in the meiosis of the Lessingianthus species which could be for two reasons: that they were not observed in meiosis because of the low proportion in which they were produced, or another possible origin of the irregular spores observed. Although it has not yet been documented for the tribe Vernonieae, it is possible that meiotic restitution at the second division originated the diploid cell of the triads and dyads observed in the species in this study. Meiotic restitution is a mechanism of unreduced gamete production extensively documented in various plant species and under different stress conditions (Pecrix et al., 2011; De Storme et al., 2012; De Storme & Geelen, 2013, 2020; Kovalsky et al., 2014). It can occur in the first and second meiotic divisions: in the first case, the pairing and subsequent segregation of the homologous chromosomes of the first meiotic division does not occur, whereas in the second case the segregation of sister chromatids occurs in anaphase II but subsequently the nucleus is restored (Kovalsky et al., 2014). The restored nucleus forms diploid gametes, which are larger than the reduced nuclei and this is visibly detectable in the spore and pollen grain at the end of sporogenesis due to its prominent size (Kovalsky & Solís Neffa, 2012). Maybe, this will constitute the first report of restitution nuclei for the tribe.
The high percentage of regular meiotic behavior and tetrad formation observed in the diploid and polyploid species studied allow us to state that both had high meiotic regularity and therefore formed normal viable gametes independently of the ploidy level. Viable unreduced male gametes might be capable of fertilizing a reduced or unreduced embryo sac which promotes sexual polyploidization in naturally hybridizing plant populations (Kovalsky et al., 2014). At the same time, sexual polyploidization leads to cytotypic diversification, which has been extensively documented for the genus Lessingianthus (Jones, 1979; Turner et al., 1979; Galiano & Hunziker, 1987; Ruas et al., 1991; Dematteis, 1996, 1997, 1998, 2002; Dematteis & Fernández, 2000; Dematteis et al., 2007; Oliveira et al., 2007a, 2007b; Angulo & Dematteis, 2012). In this sense, polyploidy played, and in fact is actually playing, an important role in the diversification and evolution of the genus. We inferred that this major force of evolution is operating through the identified mechanisms of unreduced gamete formation in Lessingianthus species, such as chromosomes out of plate, bridges without fragments and asynchrony of meiosis I and II. Meiotic restitution could constitute another mechanism of diploid gamete formation, however this has yet to be confirmed.
Pollen grain viability
In the genus, pollen grain stainability was only recorded for the natural tetraploid populations of L. plantaginodes (Pérez et al., 2021). So far, other species of the genus have not been studied.
Different staining techniques of mature pollen grain are useful and commonly used to observe the abnormalities during the development of MMC and to infer male sterility in cytogenetic studies (Marks, 1954; Alexander, 1980; Bolat & Pirlak, 1999; Rodriguez-Riano & Dafni, 2000; Peterson et al., 2010). This estimation is a relative value of the number of unstained pollen grains (depending on the technique considered) or micro grains to double sized pollen grains called “jumbo” (Taschetto & Pagliarini, 2003). According to Dafni & Firmage (1999) there is no one best test to examine pollen viability in vitro, therefore the authors recommended the use of different tests to reflect different components of pollen performance. In this study we used the meiotic behavior and tetrad formation analysis together with the technique of Pittenger & Frolik (1951) to infer pollen viability. It should be noted that pollen stainability is used in this study as an indirect measure of pollen viability that allows us to have a broader view of male fertility. Taking this into account we have noticed that despite the high ploidy levels of the species studied and the non-viable pollen grains scored, the pollen grains of the species were highly viable. Most of the species studied showed a high proportion of pollen viability, up to 70%.
Lessingianthus bardanoides is a particular case: it is a diploid species with the lowest pollen viability and therefore high sterility. Studies on its meiotic behavior could help to elucidate the origin of the abnormal pollen grains. Unfortunately, the meiosis of this species could not be analyzed. The high sterility of the pollen grains, inferred through the stainability, may interfere with the reproduction of the species and its maintenance in the population. A survey on the viability of the seeds of a natural population of L. bardanoides from Cerrado (Sao Paulo, Brazil) had 58% of seeds without embryos and, apart from this result, Cury et al. (2010) remarked on the high seed germination rate and speculated that this species is mainly propagated by sexual reproduction through seeds. Abnormal pollen grains are sterile in many cases, but in a few cases they survive, reach the female gamete and fertilize them, promoting the formation of new cytotypes and polyploid populations (Kreiner et al., 2017). Due to the low pollen viability rate estimated in this study, we were able to infer that the seeds of the L. bardanoides population studied by Cury et al. (2010) may have polyploid embryos or be of apomictic origin. Embryological studies of the original Brazilian Cerrado population studied by the authors may help to elucidate the reproductive biology of the species.
A recent study carried out by Pérez et al. (2021) reported the high pollen grain viability of L. plantaginodes, inferred up to 97%. This estimation is in accordance with our results of other Lessingianthus species and may indicate the high stability of the male gametophyte development. The high percentages of regular behavior, the high production of normal tetrads together with the high pollen viability inferred denotes that the high ploidy levels are not an apparent obstacle during male gamete formation of the studied species.
CONCLUSIONS
The study of the male gametophyte development significantly increased the scarce data on male embryology, not only for the genus but also for the tribe as well. The anatomical analysis performed allowed us to determine that polyploidy does not affect the anther wall development. The main features like tetrasporangiate anthers, four-layered anther wall of dicotyledonous development, with persistent epidermis, invasive tapetum, tetrahedral tetrads, and 3-celled pollen grains are of taxonomic relevance for the tribe. These features broadened the male cytoembryological knowledge of the genus and suggest that embryological characters related to male gametophyte development are conserved among the studied species.
We analyzed for the first time the mechanisms of unreduced male gamete production; we also studied the meiotic behavior and sporad production of Lessingianthus species and inferred the pollen grain viability. With these data, we estimated the male fertility of diploid species and compared it with polyploid species. The results demonstrated that the meiotic behavior is highly regular among the studied species. We observed that there are no differences in fertility between the diploid and polyploid species: both have high meiotic regularity. Therefore, the ploidy level is not relevant in the meiotic behavior of the analyzed species. In fact, the ploidy level did not affect the production of viable pollen grains, and consequently the male fertility is relatively high. This is clearly evident in the meiosis of decaploid L. teyucuarensis. In contrast, the highly irregular meiotic behavior of L. argenteus may strongly indicate the presence of a neopolyploid species.
In addition to the highly regular meiotic behavior, the species studied presented different degrees of meiotic irregularity at the different phases observed. In diploid specimens the irregularities were concentrated in anaphase of the first meiotic division, whereas in polyploid specimens the irregularities were dispersed throughout the second meiotic division. The main abnormal behavior identified, and therefore the principal mechanisms of unreduced microspore formation, were chromosomes out of plate, bridges without fragments and asynchrony of meiosis I and II. There is a possibility that meiotic restitution could constitute another mechanism of diploid gamete formation, but this it has to be confirmed. Given that meiosis I had the highest proportion of abnormalities we inferred that this phase is the most unstable, especially the first segregation phase called anaphase I which had bridges without fragments, asynchrony and chromosomes out of plate.
Next step in understanding the evolutionary history of the genus from its roots, is to study the development of the female gametophyte to determine whether if: unreduced embryo sacs are formed, unreduced female egg formation mechanisms are employed and which ones are, and finally if the female fertility is associated to ploidy level. With this information we will be able to shed some light on polyploid formation and set up the main basis for the study of population genetics in the genus.