Introduction
The prevalence of infections in crops has increased considerably lately, affecting harvests around the world. Among all biotic threats, fungi and oomycetes are the ones that pose the greatest risk to global food security. Diverse factors are involved in this phenomenon, which include changing climate conditions and agricultural practices. Higher temperatures in mild winters allow pathogens survival and expansion to new areas, and accelerate their life cycle (Bebber & Gurr, 2015). Many of these tropical fungal pathogens are expanding to subtropical areas, setting new threats to agricultural production.
The Ascomycete Colletotrichum graminicola (Ces.) Wils. (Glomerellaceae, Glomerellales) is recognized in Argentina as an important pathogen in maize (Zea mays L.), causing anthracnose and stalk rot (Díaz et al., 2012). This disease is relatively new in the country: during the 1970’s was considered only as a sorghum pathogen (Fernández-Valiela, 1979). C. graminicola, as initially described by Wilson, was a single cosmopolitan species with a very wide host range among grasses. However, improved understanding of C. graminicola taxonomy has confirmed that the fungus is specific to Z. mays (Belisário et al., 2022). According to Dean et al. (2012), virtually every crop grown is susceptible to at least one species of Colletotrichum spp. These fungi cause anthracnose spots and blights on aerial plant parts and post-harvest rots, generating major losses to economically important crops. Anthracnose in maize causes important yield losses, because it prevents the kernel's development or damages the stems before harvest. No-till farming techniques without a proper crop rotation have also increased the incidence and severity of maize anthracnose in both North and South America (da Costa et al., 2014). Estimates of yield reductions are as high as 10 to 20% worldwide (Belisário et al., 2022). In nearby countries such as Brazil, this disease has been responsible for most yield losses of maize (Sukno et al., 2008). C. graminicola has been reported as one of the most frequently found fungal pathogens in Argentina recently; specially affecting second season maize crops (De Rossi et al., 2016).
Cell wall degrading enzymes (CWDEs) allowed host penetration and colonization, and are utilized to obtain nutrients from plant polymers. These enzymes include ligninases, pectinases, cellulases, hemicellulases and various other hydrolases that target the cell wall polymers (Kubicek et al., 2014). The amount and variety of enzymes released depends on the pathogen’s lifestyle. Most necrotrophs discharge copious amounts of enzymes, in an attempt to degrade as much as possible before the plant is able to stand an effective defence; while biotrophs secrete relatively few CWDEs, thus operating by stealth and minimizing host damage. This difference in enzyme production is also reflected in the pathogen’s genome: biotrophs encode less CWDEs when compared to other necrotrophic pathogens (Wang et al., 2022). C. graminicola, like most Colletotrichum spp. species, has a hemibiotrophic lifestyle. During the first stages of the disease, it lives as a biotrophic pathogen, spreading through the plant tissue while inflicting minimum damage to the host, before shifting to a more aggressive necrotrophic stage, where extended areas of the host tissue are killed (Münch et al., 2008; Torres et al., 2016). CWDEs are required for virulence in many phytopathogenic fungi (Wijesundera et al., 1989; Paccanaro et al., 2017; Ma et al., 2019). Several studies have demonstrated the importance of pectinolytic enzymes in plant invasion and symptom development (Reignault et al., 2008; Armesto et al., 2019). Ramos et al. (2010) showed that the disparities in the production of polymethylgalacturonase, polygalacturonase and pectin lyase activities by C. truncatum isolates (the causal agent of soybean anthracnose) were not related with fungal growth or geographical origin, but instead were associated with differences in virulence among isolates. Ligninolytic oxidative enzymes such as laccase also play an important role in tissue maceration, as lignin is a complex aromatic polymer that hinders enzyme diffusion and can alter the extent of the enzymatic degradation of other cell wall polymers. These enzymes also show a protective effect on the phytopathogen, as they are able to detoxify phytoalexins and other phenolic compounds that are involved in host’s defence mechanism (Vetchinkina et al, 2022).
Considering that maize anthracnose is a relatively new disease in Argentina, a better understanding of the physiological behaviour of local isolates of this fungal pathogen is key to prevent and/or treat the disease, and will contribute to an ecologically sustainable integrated management. In this study, we analysed the intraspecific variability of eight native isolates of C. graminicola (obtained from symptomatic maize plants collected in different production sites in Argentina), related to growth and activity of different extracellular CWDEs putatively involved in fungal penetration and colonization.
Materials and Methods
Fungi
The isolates of C. graminicola were obtained from lesions of symptomatic maize plants. They were collected from eight Argentinean localities in the provinces of Buenos Aires [CG 4(6) (Arrecifes 34° 04' 00" S, 60° 07' 00" W), CG20 (Rojas 34° 11' 00" S, 60° 44' 00" W), CG21 (Bolivar 36° 15' S, 61° 06' W) CG22(1) CG22(2), CG23(4) and CG27 (Pergamino 33° 53' 01" S, 60° 34' 01" W)] and Santa Fe [CG25(5) (Caseros 33° 03' 00" S, 61° 10' 00" W)]. The isolates are deposited at the Phytopathology Department, Agronomy Faculty, University of Buenos Aires, Buenos Aires, Argentina.
Culture media
Pectin culture (PEC) and Galhaup (GAL) media were used. Both media shared a basal medium consisting of: MgSO4.7H2O, 0.5 g; HK2PO4, 0.6 g; H2KPO4, 0.5 g; CuSO4.5H2O, 0.4 mg; MnCl2.4H2O 0.09 mg; H3BO3, 0.07 mg; NaMoO4.2H2O, 0.02 mg; ZnCl2, 2.5 mg; ZnCl2 2.5 mg; FeCl3 1.0 mg; biotin 5 pg; thiamine 100 pg; distilled water up to 1000 ml. For PEC, pectin from apple (10 g/l) and asparagine monohydrate (4 g/l) were added as carbon and nitrogen sources respectively, and additionally 0.5 g/l of MgSO4 and 0.4 mg/l of CuSO4 were supplemented to the basal culture medium (Ramos et al., 2010). For GAL, glucose (40 g/l) was used as carbon source, and 5 g/l of yeast extract, 5 g/l peptone, 0.25 g/l CuSO4 and 1 g/l SO4Mg were added to the basal culture medium (modified from Galhaup et al., 2002).
Culture conditions
Erlenmeyer flasks (125 ml) with 25 ml of medium were inoculated with one agar plug (0.25 cm2) cut out from a colony grown on Malt Extract
Agar (MEA). Incubation was carried out at 23 °C under stationary conditions. Cultures were harvested at different incubation periods, filtered through a filter paper using a Buchner funnel and dried for 24 h at 70 °C. Dry weight of mycelium was then determined. The culture supernatants were used as enzyme sources.
Enzyme assays
Polymethylgalacturonase (PMG) and polygalacturonase (PG) (endo and exo activities) were assayed using 0.1% apple pectin or polygalacturonic acid respectively, in 50 mM sodium acetate buffer (pH 4.8) at 30 °C. Liberated reducing sugars were quantified by the Somogyi-Nelson method (Somogyi, 1952). One unit of enzymatic activity was defined as the amount of enzyme releasing 1 pmol of galacturonic acid per min. Laccase activity was measured using 2,6-dimetoxyphenol (DMP) 5 mM in 0.1 M sodium acetate buffer (pH 3.6) at 30 °C (Paszczynski & Crawford, 1991). Oxidation of DMP was determined by the increase in absorbance at 469 nm (e469 = 27 mM-cm-1). p-glucosidase was determined in 50 mM sodium acetate buffer (pH 4.8) at 30 °C, by measuring ^-nitrophenol released from 0.02% />-nitrophenyl p-D-glucopyranoside (Wood & Bhat, 1988). Enzyme activities were expressed in International Units (U) as the amount of enzyme required to release 1 pmol of product in 1 min.
Statistical analysis
The data presented in graphics was analysed using Graph Pad Prism 9.4 software. Two way ANOVA tests were performed and the differences between treatments were compared by Tukey’s multiple comparison tests (¿><0.05).
Results and Discussion
Kinetics of growth (Fig. 1) and in vitro production of several extracellular CWDEs (Fig. 2) by eight fungal isolates of C. graminicola was characterized in two liquid culture media (PEC and GAL) based on pectin and glucose as carbon sources, respectively. A two-way ANOVA analysis revealed a significant effect of isolate, medium and interaction between factors (¿><0.05) for each enzyme studied.

Fig. 1: Kinetics of growth of C. graminicola isolates. A: in PEC media. B: in GAL media. Each point represents the mean of three replicate experiments, error bars denote SEM.
All isolates were able to grow in both media, showing maxima between 8 and 10 days. More biomass was obtained in GAL (Fig. 1B) than in PEC medium (Fig. 1A) (approx. 21 mg/ml vs. 3 mg/ml), probably due to the more easily assimilable carbon source. Valúes of biomass yield attained in PEC medium were similar to those obtained when culturing C. truncatum in the same medium (Ramos et al., 2010).
No pectinolytic activity production was detected in PEC medium for some strains (Fig. 2A-B). Among CWDEs, those with pectinolytic activity have been the most extensively studied for their role in host-pathogen interactions and disease development. Pectinases in their multiple forms have proved to be important for the infection process, since they are the first polysaccharidases to be induced when fungi are grown on isolated plant cell walls, and are the first produced in infected tissue (Kikot et al., 2009; Armesto et al., 2019). The disruption of pectinase genes reduces the virulence of fungi such as Botrytis cinerea, Nectria haematococca, Penicillium digitatum and Aspergillus niger, thus hampering their pathogenicity (Have et al., 1998; Rogers et al., 2000; López-Pérez et al., 2015; Liu et al., 2017). In this study, seven out of the eight isolates showed PMG activity in GAL medium, while only four isolates presented PMG activity in PEC medium (Fig. 2A). These isolates revealed PMG activities earlier in PEC than in GAL medium. In addition, isolates CG20 and CG22(2) showed PG activities formerly in PEC than in GAL medium. These findings might be attributed to catabolite repression by the abundant reducing sugars detected in GAL medium during the first days of incubation (data not shown). PMG activity was not detected in isolate CG22(1). Most isolates exhibited the highest activity after the day of maximum growth (up to 0.28 U/ml), on the contrary in C. truncatum, the peak of PMG activity preceded this day (Ramos et al., 2010). All isolates showed PG activity in GAL medium, while only five of them showed PG activity in PEC medium (Fig. 2B). The highest activity (0.25 U/ml), was reached in GAL medium by isolate CG22(2). Most C. graminicola isolates tested here, showed the peak of PG activity after the day of maximum growth, as it was previously observed in isolates of another phytopathogenic ascomycete Macrophomina phaseolina, grown in similar culture and medium conditions (Ramos et al., 2016). PG titres obtained by C. graminicola cultures in this work, resemble those attained by C. lindemuthianum (0.24 U/ml) (Hugouvieux et al., 1997), and Fusarium oxysporum f. sp. niveum (0.4 U/ml), while C. truncatum and F. graminearum showed higher levels of PG (1.08 U/ml and 1.53 U/ ml) (Kikot et al., 2010; Ramos et al., 2010).
All isolates showed p-glucosidase activity in both GAL and PEC media (0.01-0.045 U/ml) (Fig. 2C). As in other phytopathogenic fungi, such as Fusarium spp. (García-Maceira et al, 2000), cellulases secretion in C. graminicola occurred after the secretion of pectinases. The maximum of activity was detected after 15 days in all the isolates, when reducing sugars levels were at their lowest.
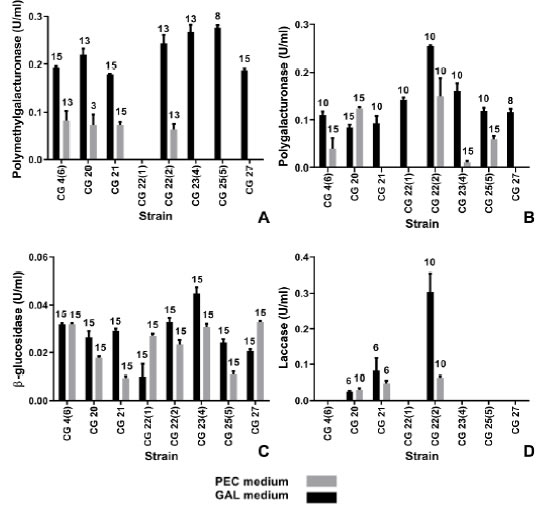
Fig. 2: Enzymatic production by C. graminicola ¡solates. A: Polymethylgalacturonase. B: Polygalacturonase. C: p-glucosidase. D: laccase, in PEC and GAL media. Numbers on top of the bars indicate the day of maximal activity, the values are the mean of three replications, error bars denote SEM.
A polyphenol oxidase characterized as laccase, was identified in the extracellular conidial mucilage of C. graminicola (Anderson & Nicholson, 1996) and might have a role in fungal survival and detoxification of plant phenols. Nevertheless, the roles of laccases in plant-pathogen interactions remain poorly understood (Levin et al, 2007), and mycelium associated laccase activity detected in this work might play a different function. Only three isolates showed laccase activity in both GAL and PEC media: CG20, CG21 and CG22(2) (Fig. 2D). The highest enzyme titres (0.063 U/ml) were
obtained in GAL medium, mostly before the day of maximum growth. Similar laccase production was detected when growing C. truncatum in a liquid medium with pectin as carbon source, six out of ten isolates produced up to 0.044 U/ml laccase (Levin et al., 2007).
The results of this study show that C. graminicola has a high intraspecific variability when evaluating CWDEs in vitro production (A-values were respectively of 35.10, 10.64, 25.30 and 14.02 for PMG, PG, p-glucosidase and laccase, with y>-values <0.0001), which is consistent with the high variability observed on a genetic level when assessing ISSR markers in these same isolates (Gatica et al., 2014).
The pattem of in vitro production of CWDEs likely reflects the in vivo pattem of enzyme production, and their role in the pathogenic process: initial pectinolytic enzyme secretion being responsible for allowing access to other cell wall components, while subsequent cellulase activity contributing to cell lysis and tissue maceration. Similar pattems were described in other phytopathogenic fungi (Ten Have et al, 2002; Kikot et al., 2009). Laccase activity could contribute to cell wall degradation in lignified tissues and protect against plant defence mechanisms. Additional work on the characterization of transcription and secretion of CWDEs in different phytopathogenic fungi will contribute to finding new specific targets to protect plants and accelerate the development of new agents for battling these fungi (Kubicek et al, 2014).
The assayed isolates of C. graminicola displayed notable differences in CWDEs production, not associated with a differential growth. This suggests a large intraspecific variability, which might be considered when choosing a method to deal with this pathogen. The aim of forthcoming studies will be to test the hypothesis that differences in aggressiveness among C. graminicola isolates causing anthracnose in maize are related with disparities in CWDE production.
Author Contributions
PN and IC designed and performed the laboratory experiments. PN and LL wrote the manuscript. All authors have read and approved the final version of the manuscript.
Acknowledgments
To Consejo Nacional de Investigaciones Científicas y Técnicas (CONICET) (PIP 11220170100283 to LL) and Universidad de Buenos Aires (UBA) (UBACYT 2020020170100163 to LL) for financial support, Dra. Marcela Gally for providing the fungal isolates and Victoria Di Stefano, Olivia Morris Hanon and Dr. Raúl Itria for their assistance in this project and comments on the manuscript.
Recibido: 19 Oct 2022
Aceptado: 24 Abr 2023