Introduction
Table grape quality influences consumer decision and sale prices. This quality is related to bunch architecture, berry size and firmness. The quality of cultivars that naturally set straggly bunches can be improved by physical and chemical manipulations with plant growth regulators (PGR; 10). Bunch compactness is modified by PGRs, affecting number and size of berries and their spatial arrangement through rachis architecture 32. In the table grape industry, gibberellic acid (GA3) is one of the most used PGR for bunch thinning, decreasing berry set and increasing berry size 9,24,29, especially in seedless table grape cultivars that naturally set compact bunches with small berries.
GA3 is used alone or mixed with other PGRs such as cytokinins and auxins 31. However, not all the effects reported for GA3 are beneficial, since it can decrease 9,22 and inhibit 24 bud fruitfulness in the season following its application. Reduction in berry skin coloration and increased occurrence of bunches with berries that differ greatly in size and maturity at harvest, known as “millerandage”, are reported among GA3 detrimental effects 2,9. In addition, GA3 applications have other adverse effects, such as increased incidence of berry drop 15. Another PGR widely used to increase bunch quality in table grapes is CPPU (Forchlorfenuron; 34), and although it is not synthesized by plants, it is a cytokinin that mimics GA3 and produces positive results without affecting bud fertility. An additional tool for table grapes production is the use of biostimulants, products of diverse biological origin containing a mixture of PGRs, but with incomplete identified composition of microbial species that produce PGRs or regulate their content in plant tissues 3,28. In Argentina, these products are registered but no specification regarding their use on vines is available (https://www.argentina.gob.ar/produccion-organica/listado/oficial-de-insumos-comerciales).
In search for an alternative to GA3, a preliminary study compared the effects of PGRs synthesized by plants on bunch quality and crop yield using GA3, 6-benzylaminopurine (BAP), indole-3-butyric acid (IBA) and abscisic acid (ABA) on Vitis vinifera L. cv. Superior Seedless. The results of this experiment showed a positive effect on bunch quality with IBA application. Therefore, further experiments were performed to evaluate the performance of IBA on bunch quality as an alternative to GA3.
Normally, the initial stimulus for fruit growth begins with pollination and correlates with elevated levels of auxins, mainly indole-3-acetic acid (IAA; 4). After fertilization, fruit growth depends on auxins produced in developing seeds, through the endosperm at the initial stages and the developing embryo during the later stages 30. Coombe (1960) evaluated changes in auxins during fruit development (from anthesis to maturity) on seeded (Muscat, Emperor) and seedless (Sultanina, Emperor Seedless and Corinth) grape cultivars. In general, auxins rise from low levels after anthesis to high levels a few weeks later, and are maintained for long periods in seeded cultivars. Costantini et al. (2007) demonstrated that auxin signal transduction allows fruit set in the absence of pollination (parthenocarpy), and that auxins enhance fertility. In addition, auxins strongly modulate growth 17 via cell division, cell expansion 13 and vascular development 1,21.
The main auxin in higher plants is IAA, which is synthesized in meristems, young leaves and developing fruit and seeds, but there are other natural molecules with auxin activity. IBA is a natural auxin synthesized by higher plants, commonly used for rooting and micropropagation 12. It has also been shown as more effective than IAA in lateral root formation 14, being considerably less expensive. At present, few publications have reported the effect of IBA on grape bunch quality and yield, and these studies have been performed with PGRs blends containing IBA, that is mixed with cytokinins, gibberellins and auxins 31.
Against the above framework, the hypothesis is that IBA sprayed to commercial vineyards improves fruit set, the number of berries per bunch, fruit growth, bunch quality and consequently yield in table grape.
Superior Seedless, also known as Sugraone, is one of the main white seedless table grapes worldwide and the most exported from the Province of San Juan, Argentina, with a cultivated area of 2359.6 ha 18. It usually has very low fruitfulness 26, with stenospermocarpic berries (i.e. the embryos begin their development after fertilization but abort at an early developmental stage, leaving a seed trace; 5), implying that the cause relies on the fact that one of the main auxin sources, the embryo, is absent.
The aim of this study was to examine exogenously applied IBA as an alternative tool to improve seedless table grapes yield and quality indicators. The effect of IBA, applied at different dosages and phenological stages, was evaluated in comparison with GA3 on Superior Seedless bunch structure, yield and quality. Furthermore, an anatomical analysis was performed to explain PGRs effects on bunch architecture. After the first preliminary experiment and with IBA showing the best results, a second growing season evaluated and determined the most effective dose and adequate phenological stage.
Material and methods
Plant material and treatments
The trial was conducted over two seasons: 2012-2013 and 2014-2015 in a comercial Vitis vinifera L. cv. Superior Seedless vineyard, located at Carpintería, San Juan, Argentina (31°43'S and 68°35'W; 642 m a.s.l.). Grapevines were planted in 2005, own rooted, spaced at 2.5 x 2.5 m, trained on a local trellis system called “Parral “or overhead. The soil of the experimental site was sandy with 60-80% stone content. The vineyard was drip irrigated. Vines were cane pruned with 10 canes per vine and 12 buds each. When inflorescences were developed [phenological stage 13 6], vines were thinned to 30 bunches per plant. Only well-developed basal clusters were left. A completely randomized design with 5 replicates, with one vine as experimental unit, was selected according to vigor homogeneity (trunk diameter). During 2012-2013, three doses of IBA and GA3 (2, 20 and 50 ppm) were separately sprayed at three phenological stages 6; 15 cm shoot length (Stage 13), full Bloom (Stage 25-26) and berries pea size (Stage 31). Commercial IBA (SIGEL, GEO SRL, Argentina) and GA3 (10%, GIBERELINA KA, S. Ando & Cía. SA, Argentina) were dissolved in water, with 0.1% (v/v) of Triton X-100 as surfactant and a minimum volume of 96% aqueous ethanol. A solution containing water with the concentration of surfactant and ethanol as described above was used as control. Treatments were applied with a backpack sprayer to the whole vine until runoff (ca. 100 mL plant-1). Applications were done in the late afternoon to avoid photo degradation and to prevent the solution from fast drying off by high temperatures. In the second season, three IBA doses (2, 20 and 50 ppm) and 4 phenological stages, 15 cm shoot length (Stage 13), full bloom (Stage 25-26), fruit set (Stage 26-27) and berries pea size (Stage 31) were evaluated.
Yield components
Four bunches per plant were selected and used as observational units (20 bunches per treatment). After berry softening phase (Stage 36, Coombe 1995), fruit ripening was weekly monitored by measuring accumulation of total soluble solids (TSS) with a hand-held refractometer (Pocket PAL-1, Atago®, Tokyo, Japan). All the treatments were harvested when the control treatment reached 14 to 15°Brix. The collected bunches per experimental unit were placed in plastic bags and kept in ice to prevent dehydration. Bunch fresh weight (FW), Berry FW and berry size (diameter and length) from 20 randomly selected berries per bunch were determined. Berry number was calculated based on bunch and berry weights.
Bunch architecture and Anatomical analysis
Rachis FW was registered immediately after berry removal. After that, bunch structure was evaluated by measuring length and diameter of the central rachis, lateral rachis ramifications (4 upper lateral) and pedicels (at the insertion point of the berry) from 6 randomly selected pedicels. All diameters were measured with a digital caliper. In the second season bunch compactness was assessed by ascertaining the distance between rachis laterals (internodes) and berries number per cm of 4 upper lateral ramifications (Figure 1).

Rachis length and diameter (Ø Rachis), lateral rachis length and diameter (Ø Lateral), pedicel length and diameter (Ø Pedicel), bunch rachis lateral distance (Internode) and number of berries per cm of upper lateral rachis (#Berries cm-1). Longitud y diámetro de raquis (Ø Rachis), longitud y diámetro de raquis laterales (Ø Lateral), longitud y diámetro de pedicelos (Ø Pedicel), distancia entre raquis secundarios (internado) y número de bayas por cm en raquis laterales superiores (#Berries cm-1).
Figura 1: Esquema de la arquitectura de racimos.
Samples for anatomical observations were collected from bunches treated at full Bloom with IBA 20 ppm, GA3 20 ppm and control plants. Histological micro-sections (13 μm) of the rachis (2 cm above the upper lateral rachis) were prepared using a rotary standard microtome, stained, and evaluated with a microscope (Model 16, Carl Zeiss, Gottingen, Germany). Photomicrographs were taken and digitalized with a camera (AxioCamHRc, Carl Zeiss) according to the protocol of Travaglia et al. (2012). Xylem and phloem areas were calculated using the software Image Pro-Plus (Media Cibernetics Inc, Rockville, MD).
Postharvest quality indicators
After harvest, 4 bunches per experimental unit were packed. Each bunch was individually bagged in perforated polyethylene and packed in carton boxes with a SO2 generator pad (fast and slow-release phases; 1 g Kg−1 of fruit) to prevent decay. The packages were air cooled to 4 °C for 9 hours. Then, these packed bunches were cold stored for 60 days at 0°C and 95% relative humidity for post-harvest evaluation. Rachis browning, berry shatter and berry firmness were evaluated after cold storage. The rachis of each bunch was visually evaluated with a 1-4 scale where: 1 = healthy, entire rachis including pedicels are green and healthy; 2 = slight, rachis in good condition, but pedicel browning is noticeable; 3 = moderate, browning is visible in pedicels and secondary rachis; 4 = severe, pedicels, secondary and primary rachis are completely brown 8. Percentage of berry shattering (loss of berries from the cap stem) was calculated based on detached berries and total number of berries per bunch. Berry firmness was measured on the equatorial position of 20 randomly selected berries per bunch with a penetrometer (Fruit Pressure Tester, Model FT 327, Italy).
Statistical analysis
The analyses were performed with InfoStat Software (InfoStat version 2009; Grupo InfoStat, Córdoba, Argentina) using generalized linear models. Means were separated using LSD Fisher test with a level of significance P≤0.05. Principal component analysis (PCA) was performed using biplot graphics and standardized (centered and variance-scaled) data (InfoStat version 2009; Grupo InfoStat, Córdoba, Argentina).
Results
Yield components
During season 2012-2013, bunch FW, berry FW, berry diameter and berry length were increased in almost all IBA and GA3 treatments as compared to control (Table 1, page 168).
The most prominent effect on bunch FW was obtained with IBA applied at full bloom, in a dosage dependent manner and with an increase of up to 84% as compared to the control. Furthermore, berry FW with IBA at full bloom, increased 37 to 50% more than the control. The number of berries per bunch decreased by 33% under GA3 50 ppm and increased by 21 to 28% at all doses of IBA, both applied at full bloom.
Treatments did not affect TSS, except for GA3 50 ppm at full bloom, which showed higher TSS than any other treatment.
In the 2014-2015 season, bunch FW, berry FW and berry size were increased by IBA treatments as compared to control (Table 1, page 168). However, during the second season, IBA applied at fruit set produced the highest bunch FW and number of berries per bunch, increasing 60% and 30 to 46% respectively, with respect to control (Table 1, page 168). In addition, this treatment did not delay ripening, which achieved the same TSS concentration as the control. Even though the treatments IBA 2 and 50 ppm at shoot 15 cm, IBA at full bloom (all doses), and IBA 50 ppm at berries pea size showed significant difference with IBA at fruit set and the control, all treatments achieved commercial maturity (14 to 15 °Brix) (Table 1, page 168).
In both seasons, all treatments increased berry diameter and length, by 1 to 2 mm and 3 to 4 mm respectively, compared to control, without affecting the typical oval shape of Superior Seedless berries (berry diameter to length berry ratio; Table 1, page 168).
A seasonal effect was also observed, e.g. during 2014-2015, bunch FW and berry FW in control were 24% lower than in 2012-2013; however, differences among the treatments with PGRs still occurred, and similar trends between treatments and control could be observed.
Bunch architecture and Anatomical analysis
Table 2 (page 169) indicates PGR effects on bunch structure.
During the 2012-2013 season, the highest rachis elongations were observed with GA3 at 50 ppm applied at 15 cm shoot length. However, this treatment showed twisted rachis (overdose symptoms; Figure 1S). An increase in pedicel diameter occurred with 2 ppm GA3 application at 15 cm shoot length, full bloom (all doses), and berry pea size (2 and 50 ppm). All the treatments with PGRs resulted in an increase in pedicel length. IBA at full bloom (all doses) showed the highest lateral length, the highest rachis diameter and rachis FW.
In the 2014-2015 season, all the IBA treatment increased the rachis FW, lateral rachis length, rachis diameter and the distance between lateral ramifications (rachis internode). In addition, IBA treatments, except for the lower dosages at berry pea size, achieved the highest lateral rachis diameter. The number of berries per cm was increased by IBA at 15 cm shoot length (20 and 50 ppm), and fruit set (50 ppm). Pedicel diameters were not affected by the treatments (Table 2, page 169).
The anatomical analysis showed that the application of IBA increased the vascular áreas in the basal section with respect to the control and GA3 treatments. In particular, the xylem and phloem areas were increased by IBA 20 ppm applied at full bloom, 59% and 27% respectively, as compared to the control (Figure 2).
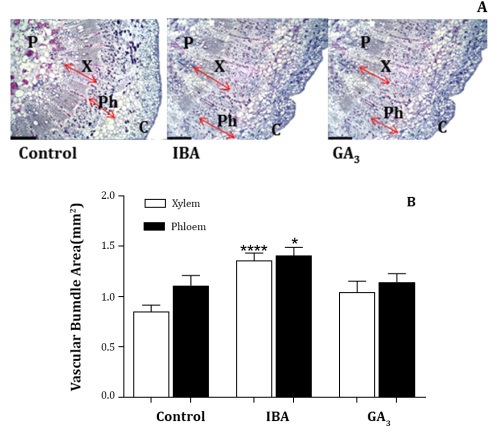
Samples were prepared using a rotary standard microtome, stained and evaluated with a microscope (Model 16, Carl Zeiss, Gottingen, Germany), and photomicrographs were taken and digitalized with a camera (AxioCamHRc, Carl Zeiss). a: Microphotographs of rachis histological anatomy, scale bar 400 μm. X: xylem, Ph: phloem, p: pith, C: cortex. b: Total vascular, xylem and phloem área (mm2 per vascular bundle). Asterisks denote significant differences from the control according to Fisher´s LSD test (*, P≤0.05; ***, P≤0.001). Las muestras se prepararon utilizando un micrótomo estándar rotatorio, se tiñeron y evaluaron con un microscopio (Modelo 16, Carl Zeiss, Gottingen, Alemania), tomando y digitalizando microfotografías con una cámara (AxioCamHRc, Carl Zeiss). a: Microfotografías de la anatomía histológica del raquis, barra de escala 400 μm. X: xilema, Ph: floema, p: médula, C: corteza. b: Área vascular total, xilema y floema (mm2 por haz vascular). Los asteriscos indican diferencias significativas con respecto al control según la prueba LSD de Fisher (*, P≤0,05; ***, P≤0,001)
Figura 2: Secciones transversales (13 μm) del raquis, 2 cm por encima del raquis lateral superior en cosecha, en plantas de Vitis vinifera L. cv. Superior Seedless, tratadas con IBA 20 ppm y GA3 20 ppm en plena floración y control.
Phloem area showed larger than xylem area, especially in the control, with a reduced xylem to phloem ratio (Figure 2).
The PCA analysis shows that the xylem and the phloem areas, bunch FW, berry FW, Berry size and the number of berries per bunch were associated with IBA (all doses); markedly for IBA 20 ppm at full bloom (Figure 3, page 171).
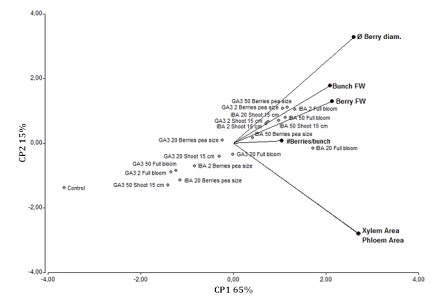
Bunch fresh weight (FW), berry FW, number of berries per bunch (#Berries), berry diameter; and the xylem and phloem areas, in the different IBA and GA3 treatments and controls, season 2012/2013. Peso fresco (FW) del racimo, FW de la baya, número de bayas por racimo (#Berries), diámetro de la baya; y áreas de xilema y floema en los diferentes tratamientos IBA, GA3 y control, temporada 2012/2013.
Figura 3: Biplot del análisis de componentes principales (PCA) de componentes del rendimiento.
Table 3 (page 171) shows that the post-harvest quality was improved by IBA and GA3 treatments.
Table 3: Bunch quality at postharvest: rachis browning, berry shatter and berry firmness of Vitis vinifera L. cv. Superior Seedless plants treated with GA3 and IBA vs. control, season 2012/2013 and 2014/2015. PGRs were applied at different phenological stages and doses.

Rachis browning was reduced by applications of IBA consistently during both growing seasons. During the first season, browning was reduced by IBA at full bloom (all doses) and at berry pea size (20 ppm), while in the second season it was reduced by all IBA treatments at full bloom, fruit set and berry pea size. In addition, these treatments showed the highest values of rachis thickness at harvest (Table 2, page 169).
Berry shatter was less than 1% during both seasons in bunches treated with IBA (all doses). On the other hand, GA3 (20 and 50 ppm) at full bloom increased shatter in more tan 1%, in correlation with thicker pedicels (Table 2, page 169).
Post-harvest berry firmness was increased by both PGRs, as compared to control and in correspondence with berry size at harvest (Table 1, page 168).
Discussion
The yield components, bunch architecture and compactness of Vitis vinifera cv. Superior seedless were modified by IBA treatments. An increased number of berries per bunch achieved by IBA applied at full bloom and fruit set, is in correspondence with previous findings where IAA increased fruit set. In fact, Costantini et al. (2007) found that ‘Thompson Seedless’ and ‘Silcora’ (both varieties genetically engineered with an ovule-specific auxin-synthesizing gen (DefH9-iaaM), that increased IAA levels in flowers and fruits) had higher fertility and increased yield, with 30% and 15% increase in berry number, respectively.
Tecchio et al. (2005) also found that IBA applied at 50 ppm mixed with kinetin (cytokinin) and GA3 applied 15 days after full bloom on ‘Tieta’ (Suffolk Red Seedless) increased berry number per bunch. The current results suggest that deficiencies in endogenous auxins associated with embryo abortion at early stages of development in Superior Seedless (stenospermoscarpic fruits) can be corrected by exogenous IBA applications.
Most significantly, bunch FW, berry FW and the number of berries per bunch were increased by IBA treatments at full bloom and fruit set, in association with higher rachis vascular tissue area. In addition, those treatments did not generate bunch compactness, since rachis internode and lateral length also augmented. These effects correlate with the reported role of auxins in promoting cell division, cell expansion 13 and vascular development 21. Guzmán et al. (2021) reported an increase in the total vascular bundle área (xylem and phloem) and rachis hydraulic conductivity with IBA plus GA3 application at full bloom in Superior Seedless. Xylem to phloem ratios are affected by auxin concentration. High auxin concentrations induce xylem as well as phloem, but at low auxin concentrations, only phloem differentiation occurs 1, possibly explaining the reduced xylem to phloem ratio of our control treatment. Furthermore, Else et al. (2004) reported an increase in the production of vascular tissues in rachis and pedicels dependent of polar auxin transport through pedicels in wild cherry (Prunus avium L.) allowing the fruit to get more water and nutrients. This could explain how, even though the number of berries was increased, TSS were not affected by IBA treatment. During the first season, GA3 50 ppm at full bloom showed higher TSS than any other treatment. This result may depend on the smaller number of berries per bunch in this treatment, (33% and 46% fewer berries than the control and IBA treatments at full bloom, respectively).
Seasonal effects on bunch FW and berry FW were probably given by weather conditions during bunch and berries growth period. During 2014, daily mean air temperatures were higher than in 2012 (31 °C vs 28 °C), surpassing 35 °C (maximum temperature) throughout November and December. These temperatures exceed the photosynthetic optimum for grapevine (below 30 °C), affecting growth conditions. In addition, higher night-time mean air temperatures were also registered in 2014 (28 °C vs 25 °C in 2012), possibly increasing the proportion of respired assimilates 19.
Postharvest quality was augmented by IBA, since rachis fresh weight and diameter were improved in correlation with augmented vascular tissues, and reduced rachis browning. The results coincide with those obtained by Mulet et al. (2017) for Superior Seedless with application of IAA at 8-10 mm berry size (berry pea size). However, the treatment IBA at 15 cm shoot length increased rachis diameter but did not have any effect on rachis browning. A similar effect was obtained by Raban et al. (2013) with applications of GA3 and CPPU (cytokinin) in Superior Seedless, Redglobe and Crimson Seedless, where rachis thickness was increased with no browning reductions. Nevertheless, since our results were not consistent, further research is needed to clarify this finding.
Berry shatter was increased by GA3 at full bloom, in correlation with thicker pedicels. The results suggest that pedicel thickness enhances shatter, possibly given by a lesser pedicel flexibility 34. García-Rojas et al. (2018) found that GA3 applications produced an over-expression of key genes for pedicel lignification and an increase in berry drop. However, considering the fact that up to 3% of shattering is currently accepted for high quality table grape commercialization, none of the PGRs treatments represent an inconvenience since maximum shattering were under 1% 35.
Both PGRs improved berry firmness. Marzouk and Kassem (2011) reported an increase in berry firmness after application of gibberellins in the early stages of fruit growth (4-5 mm fruitlet diameter, and veraison) of Thompson Seedless, then related to berry quality. Guzmán et al. (2021) improved berries firmness and freshness appearance after 60 days cold storage with IBA plus GA3 in Superior Seedless at full bloom.
Conclusions
The results presented here support the use of IBA as an alternative to GA3, to improve yield components and bunch quality of Superior Seedless. The proposed alternative, spraying IBA at full bloom and at fruit set, enhanced bunch weight by increasing berry size and weight, in correlation with the promotion of vascular tissue area. In addition, IBA treatments increased the number of berries per bunch, without generating compactness. These results, may be as well beneficial for cultivars that naturally are prone to poor fruit set. Moreover, IBA generates positive effect on postharvest quality via reduction of rachis browning and increase of berry firmness. Finally, it is to be considered that IBA constitutes a plant growth regulator, synthesized by plants, less expensive and while lacking GA3 negative effects. It will be interesting to find out in future studies, the specific IBA doses and phenological stages application in different table grapes varieties.