Introduction
Ruminants live in symbiosis with the microorganisms that inhabit their rumen. Therefore, the nutrition of ruminants is directly related to microbial fermentation, which occurs in the reticulum-rumen 66. However, the population demographics of these microorganisms promote differences in fermentation, and therefore, the manipulation of this microbial community can be carried out in several ways 37. One is via feed additives, which can facilitate the digestion of the diet components 66. Nutritional manipulation modulating the rumen environment has direct effects on the characteristics of ruminant products 26.
Additives are, by definition, substances, microorganisms, or formulated products added inten tionally, which may or may not have nutritional value in the diet 43. Additives used in ruminant diets can be categorized as antibiotics, i.e., monensin, lasalocide, and salinomycin; prebiotics, i.e., plant extracts, tannins, exogenous enzymes, and essential oils; probiotics, i.e., yeasts 30.
Given the above, this review describes the main additives used in ruminant nutrition and discusses their effects on animal performance and animal product quality.
Ionophores
Ionophores have originally been used as coccidiostats in poultry, but later, it was observed that cattle fed chicken litter contaminated with ionophore residues showed improvement in feed efficiency due to changes in rumen fermentation 9. Ionophores are highly lipophilic polyesters with a molecular weight between 500 and 2,000 Daltons; they can accumulate in cell membranes and modify ionic transport 55 as they are able to act as transporters of different ions and protons across the phospholipid membrane of gram-pos itive and protozoan bacteria (Figure 1).
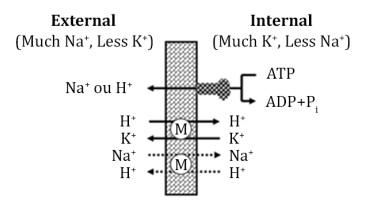
Figura 1: Mecanismo de acción de los ionóforos (M- monensin) sobre la membrana celular (adaptado de Russell & Strobel, 1989).
Due to the need of microorganisms to maintain their membrane gradient, protons must be Na+ ions, which flow into the intracellular medium and must be expelled through ATPase. However, this action is energetically costly, which raises the energy need to maintain micro organisms, compromising cell proliferation and causing bacterial death 55. In addition, the higher concentration of cations within the bacterial cell increases osmotic pressure and attracts excess water, which results in cell swelling and, consequently, bacterial lysis. The action of ionophores is generally more effective at a pH lower than the pKa value of the ionophores (monensin = 7.95), which occurs because the rumen pH favors the penetration of the bacterial cell membrane ionophore 9. Gram-positive bacteria and protozoa are more sensitive to ionophores because they do not have an outer membrane that provides protection, in contrast to gram-negative bacteria 9,55.
The use of ionophores as a feed additive is common in ruminant nutrition, especially in dairy and beef cattle. Their inclusion in diets generally causes a reduction in dry matter intake and milk production but an increased body weight gain 1. Such improvements are associated with improved energy and protein metabolism, in addition to an improved health status. The effect of ionophores on energy metabolism is closely related to their ability to facilitate the growth of gram-negative rather than gram-positive bacteria and protozoa, resulting in a lower acetate: propionate ratio, with a consequent increase in the flow of gluconeogenic precursors 2 and a lower availability of H2 ions, resulting in less energy loss via methanogenesis 1,36.
The effects of ionophores on energy metabolites have been investigated by Duffield et al. (2008) through a meta-analytical study, in which monensin supplementation reduced the serum concentrations of non-esterified fatty acids, beta-hydroxide butyrate acid, and acetoac etate in dairy cows, suggesting less mobilization of body reserves in relation to diets without monensin supplementation. The same authors stated that the supply of gluconeogenic precursors favors the improvement of the animals' energy status, reducing fat mobilization.
Appuhamy et al. (2013) performed a meta-analysis of 123 papers, covering the period 1970 to 2011, and observed that monensin supplementation reduced methane production by 7 g/day for dairy cows and 19 g/day for steers, representing a reduction in energy losses.
Other authors also reported that ionophore supplementation reduces methane production 36. In addition to the effects on methanogenesis, Appuhamy et al. (2013) also observed that supplemented animals had a lower dry matter intake and an increased milk production, indicating a higher efficiency than in non-supplemented animals. Similarly, related by Golder & Lean (2016) in meta-analytical study. Thus, in cases with beef cattle, monensin supplementation reduced dry matter intake, promoted greater weight gain, and improved feed efficiency 1,6,32,36. Duffield et al. (2008) observed that ionophore supplemen tation decreased the protein and fat percentages of milk; however, fat yield did not change, whereas protein yield was increased with ionophore supplementation. The reduction in milk fat percentage could be related to fat dilution due to an increase in milk production promoted by ionophore inclusion in the diet.
Despite the aforementioned effects on milk composition, the responses appear to be heterogeneous and may be related to the interaction of ionophores with the composition of the diet. A recent meta-analysis 25 has shown that even with greater milk production, protein and fat percentages, as well as protein and fat yield, are not affected by iono phore supplementation. The same authors also did not observe any effects on carcass fat percentage, subcutaneous fat thickness, and marbling degree in beef cattle. Lemos et al. (2016) supplemented finishing cattle with ionophores in high-concentrate diets and observed no effects on carcass characteristics.
The fatty acid profile of products from ruminants supplemented with ionophores can be changed by the ionophore effect on the bacterial population, with a consequent effect on the biohydrogenation of unsaturated fatty acids in the rumen. Changes in the fatty acid profile were observed by Duffield et al. (2008), show reported a reduction in saturated fatty acids and an increase in unsaturated fatty acids, particularly in the conjugated linoleic acid concentration of animals fed with diets containing ionophores. Despite the beneficial effects of ionophores on the fatty acid profile, such benefits are partly dependent on an adequate substrate supply (polyunsaturated fatty acids) for biohydrogenation 50.
Although ionophores bring benefits to animal production, in 2006, the European Union has banned the use of these products due to public concerns about the use of antibiotics, fearing that the bacteria would acquire resistance and compromise the health of animal product consumers when animals were supplemented with ionophores. In this sense, several other additives have been produced and tested in the feeding of ruminants, particu larly yeasts, enzymatic complexes, essential oils, plant extracts, and chitosan.
Yeasts
Against the background of the growing demand for animal products from organic production, studies have been developed to improve production and efficiency in these systems 45, highlighting the use of yeast (i.e., Saccharomyces cerevisiae).
There are two main categories of yeasts commercially available for use in animal feed, classified based on the active ingredients and their modes of action. The first category represents yeast cultures; they are not dependent on live yeasts to exert physiological effects after ingestion and provide a mixture of micronutrients composed of soluble cell contents, vitamins, minerals, proteins, peptides, amino acids, lipids, organic acids, esters and alcohols, B vitamins, polyphenols, organic acids, antioxidants, and yeast cell wall compounds such as β-glucans and mannan oligosaccharides. Although these micronutrients can stimulate bacterial growth in the rumen, increase fermentative capacity, and favor animal perfor mance; however, the effects of supplementation with yeast products have mainly been attributed to β-glucans and mannan oligosaccharides present in the yeast cell wall 4.
The second category is represented by products with live yeast cells (more than 15 billion cells/g of product), and the improvement observed with the supply of live yeasts is associated with the removal of oxygen from the rumen environment since yeasts can perform aerobic respiration. Similar to fermentation, the necessary energy for microbial growth is generated, and the intake of traces of oxygen favors the growth of strictly anaerobic bacteria, such as fiber-degrading bacteria 5. However, it is valid to point out that despite the benefits promoted by live yeasts on rumen environment, the life span of these microor ganisms in the rumen is approximately 30 hours. If the yeast population is not renewed, the concentrations of these microorganisms in the rumen are reduced to undetectable levels after 4 to 5 days 5 since the optimal pH for yeast growth is 4.5 and the ruminal pH is approximately neutral (7.0), requiring constant yeast supplementation. Based on the short lifespan of yeasts in the rumen, the effects of live yeast supplementation are similar to those of yeast culture supplementation (supply of micronutrients), indicating that the effects of both types of yeasts are similar.
The effects of yeast supplementation on several species of ruminants (cattle, buffalo, goats, and sheep) were evaluated by Desnoyers et al. (2009) in a meta-analysis including more than 157 experiments. These authors observed a greater intake and digestibility of dry matter as well as a higher concentration of volatile fatty acids and a higher pH. Similar results were observed by Poppy et al. (2012) in a meta-analysis conducted to evaluate the effects of yeast supplementation on lactating dairy cows. The authors found an increase in dry matter intake in early lactation, which is of great importance because in this period, the cows are in an energy deficit, which generally results in a decreased DMI. The authors also observed a reduction in dry matter intake in the final third of lactation, but in both cases, supplemen tation with yeast increased milk, fat and protein yields, expressed in kg/day, indicating an increased feed use efficiency.
Yeast does not directly affect rumen pH; it impacts the ruminal microflora by stimulating the growth of bacteria that use lactate, i.e., Megasphaera elsdenii, Selenomonas ruminantium13 and Anaerovibrio lipolytica5. The use of lactate as a carbon source by bacteria reduces its concentration in the rumen environment, resulting in a lower pH. This modification favors the growth of cellulolytic bacteria, causing greater fiber digestion and the production of volatile fatty acids. In addition to pH stabilization, the use of lactate as an energy source will give rise to propionate, as the final product of lactate fermentation 42, increasing the energy supply to the host animal (Figure 2).

Figura 2: Esquema propuesto para explicar los modos de acción de la levadura sobre la microbiota ruminal y sus consecuencias sobre la fermentación ruminal (Adaptado de Chaucheyras-Durand et al., 2008).
Increased cellulolytic population, fiber digestion, and the increased production of volatile fatty acids are also associated with reduced residual oxygen in the rumen envi ronment and/or with the supply of micronutrients. This has been observed by Zhu et al. (2017) when they supplied yeast products and verified an increased growth of the cellulo lytic bacterial population, mainly Ruminococus flavefaciens and Fibrobacter succinogenes. However, in this research, there were no effects of supplementation on pH, indicating that the population increase is related to the supply of micronutrients present in yeast cultures. Another important point to be considered is that the increase in the growth of bacteria in the rumen increases the flow of microbial nitrogen, implying a greater supply of amino acids and facilitating animal performance.
The improvements observed by Desnoyers et al. (2009), Poppy et al. (2012), and Rossow et al. (2018) in terms of productive characteristics were not observed in several other studies 33; these discrepancies indicate that the effects of yeast supplementation are dependent on several factors, such as the yeast strain used 54, the viability in the case of live yeasts, diet composition, and the physiological status. Overall, the mechanisms that explain the link between yeast supplementation and increased productivity are still unclear.
Fibrolytic enzymes
Enzymes are proteins acting as biological catalysts and are involved in all biological reac tions, accelerating, under specific conditions of humidity, temperature, and pH, the chemical reactions in the cells of living organisms 41. Ruminants fed with roughage are dependent on the synthesis and secretion of enzymes by bacteria, fungi, and protozoa, enabling and accelerating the fermentation of fibrous compounds present in the diet through the action of cellulases, xylanases, β-glucanases 58.
The exogenous fibrolytic enzymes used in ruminants, mainly cellulases and xylanases, refer to a class of enzymes produced by fungal (mainly Trichoderma longibrachiatum, Asper gillus niger, and A. oryzae) and bacterial sources (Bacillus spp., Penicillium funiculosum) with high cellulosic and hemicellulosic activity, which can be incorporated in liquid or granular form in the total mixed ration or separately added on roughage and concentrated feeds, as well as on supplements and mineral vitamin premix 3.
Cellulase is the most prominent group of hydrolytic enzymes and catalyzes the hydro lysis of β-1,4 bonds in cellulose. Cellulose degradation is complex and involves several specific enzymes that contribute to cellulase activity 3. In general, endocellulases (endo glucanase, endo-β-1,4-glucanase, carboxymethyl cellulase or β-1,4-glucan, and glucan hydrolase) randomly cleave internal glycosidic bonds in the cellulose chain to produce cellulose oligomers of various degrees of polymerization, whereas exocellulases (exoglu canase, exo-β-1,4-glucanase, β-1,4-cellobiosidase, cellulose) hydrolyze the cellulose chain non-reducing end, yielding cellobiose, and β-glucosidases (cellobiase or glucohydrolase) 38 hydrolyze oligomers of short-chain cellulose and cellobiose to glucose (Figure 3).

Figura 3: Representación esquemática de la degradación de la celulosa a glucosa por la acción secuencial de endoglucanasas, exoglucanasa y glicosidasas. Los asteriscos indican el punto de escisión / acción enzimática (Adaptado de Kozloski, 2017).
The effects of fibrolytic enzyme supplementation have been observed by several authors 49. For example, Arriola et al. (2011) observed that dairy cows fed low-concentrate diets supplemented with fibrolytic enzymes used the feed more efficiently than cows fed high-concentrate diets without enzyme supplementation due to an increase in ruminal pH, which reduced the risk of acidosis by increasing ruminal pH without altering the production of volatile fatty acids and milk. These authors reported an increase in feed efficiency due to a lower dry matter intake with enzyme supplementation. Lunagariya et al. (2020) reported that dry matter and nutrient intake was not influenced by enzyme supplementation. However, the authors observed an increase in feed efficiency, the digestibility of nutritional fractions, and body weight gain in non-pregnant Gir and crossbred dairy cows.
Supplementation with fibrolytic enzymes also improved the average daily gain, the use of feed nutrients and feed efficiency, explained by the greater digestion of fibrous compo nents, and resulted in an improved digestion of the fibrous fraction and in the production of volatile fatty acids 22. Chung et al. (2012) reported that enzyme supplementation increased the number of fibrolytic bacteria (Fibrobacter succinogenes) compared to that of non-fibrolytic ones (Ruminobacter amylophilus).
The increase in feed digestion is probably not simply the result of supplemental enzyme activity, whereas the contribution of exogenous enzymes added to diets to the overall rumen activity is relatively small 30. Probably, the exogenous enzymes act synergistically with the enzymes secreted by ruminal microorganisms, resulting in a more pronounced fibro lytic effect in the rumen environment 58. Concomitantly, improved digestibility is asso ciated with the breaking of physical fiber barriers during the digestion process, favoring microbial access and colonization 58, which result in a higher number of microorganisms in the diets added with enzymes 16.
However, it is important to note that while the supply of exogenous fibrolytic enzymes may increase the degradation rate and the extent of fiber degradation in the rumen 51, the absence of positive results may be related to the animals' physiological state, the supply mode, the supply amount, and the characteristics of the used enzymes 3,11. According to Adesogan et al. (2014), the exogenous fibrolytic enzyme considered ideal for improving performance must have the following characteristics: a) contain appropriate substances to increase fibrolytic activity and improve fiber digestibility; b) contain appropriate amounts of cofactors, coenzymes, and activators (when necessary) to optimize the activities of fibro lytic enzymes without inhibiting them; c) not be susceptible to degradation by ruminal microorganisms or hydrolysis by plant, ruminal, or microbial proteases; d) present a robust composition so that it does not show significant variation from one batch to another; e) be produced from fungi or bacteria that produce large amounts of enzymes, either naturally or through genetic modification; f) present optimum and stable activity under the conditions of the place where it will exert its hydrolytic effect; g) must be in liquid form or dissolve quickly and completely in water; h) be thermostable if added during the manufacture of feed; i) maintain its hydrolytic activity when properly stored for long periods; j) be considered safe for use. In addition Adesogan et al. (2014) also pointed out that additional factors could explain the variability of the results of experiments with exogenous fibrolytic enzymes, such as inadequate designs, low statistical power, and short-term experiments.
Arriola et al. (2017), in a meta-analytical study evaluating effects of supplementation with fibrolytic enzymes in diets for dairy cows, observed that the supply of exogenous enzymes did not alter the daily dry matter intake and feed efficiency but tended to increase dry matter and neutral detergent fiber digestibility. The improvement in digestibility promoted discrete increments in milk production, the production of 3.5% fat-corrected milk (kg/day) of protein, lactose, and fat; in contrast, supplementation reduced the percentage of fat milk, which is justified by the dilutive effect promoted by the greater milk production 7. In another meta-analytical study, Tirado-González et al. (2018) observed results similar to those of Arriola et al. (2017), in which enzyme supplementation provided greater milk production as well as protein and fat yields, in addition to a higher average daily gain in beef cattle.
Essential oils
In recent years, there has been a considerable interest in the use of essential oils, driven by the concerns caused by the use of ionophores, which may enable increased resistance to antibiotics. Essential oils are natural, complex, and volatile compounds with a strong odor. They are synthesized from different plants by distillation drag with water vapor or solvent extraction 20.
Essential oils can be used as additives in ruminant nutrition, modulating the rumen envi ronment similar to antibiotic additives 28. Figure 4 (page 182) illustrates the mechanism of antimicrobial action of essential oils on the bacterial cell.

Figura 4: Mecanismo de acción antimicrobiana de los aceites esenciales sobre las bacte rias (Adaptado de Burt, 2004).
Essential oils are hydrophobic but lipophilic, departing from the aqueous phase into the lipid bilayer of the cytoplasmic membrane of bacteria and accumulating and interacting with their membranes 12,17. Consequently, the membrane becomes more permeable, enabling ion translocation and, hence a decrease in the ionic gradient. Furthermore, essential oils can also interact with membrane proteins and/or other cytoplasmic components 10. To track changes in the ionic gradient, the bacteria use the ion pump to drive the ions; however, such action demands high amounts of energy, affecting bacterial growth 10. The interaction of essential oils with the cytoplasmic components can cause cytoplasmic coagulation and lead to cell lysis.
Combinations of different essential oils modify their individual effects because of the possible synergism between compounds with different functions and mechanisms of action and the interaction with other active ingredients, amplifying the effect when mixed 10. Some types of essential oils stimulate rumen fermentation, whereas others inhibit metha nogenesis, increase production, and change the profiles of volatile fatty acids and nitrogen metabolism 12.
Valero et al. (2016) observed increased feed efficiency and average daily gain in cattle supplemented with essential oils, but without changes in carcass characteristics and meat quality. Similar results were observed by Ornaghi et al. (2017), who tested two types (cinnamon and clove) and two doses (3.5 and 7.0 g/day) of essential oils in diets for Nellore cattle. These authors attributed the positive effects on the antimicrobial properties and the presence of volatile and odorous compounds from the essential oils, with a greater dry matter intake, showing an improved acceptability of the diet. An increased acceptability of diets with essential oils has also been reported by other authors 20.
Improvements in performance and feed efficiency were observed by Moura et al. (2017), who included 0.5 g/kg of dry matter in finishing lamb diets. According to the authors, copaiba essential oil had a greater antimicrobial activity toward gram-positive bacteria than gram-neg ative ones, leading to changes in the microbial population. Ornaghi et al. (2017) stated that as gram-negative bacteria are more likely to produce propionate, a gluconeogenic precursor, the availability of glucose is increased, which may justify the greater weight gain. However, such effects of essential oils on rumen microbial populations are still inconclusive 57.
The effects of essential oils were also tested by Tomkins et al. (2015), who used the commercial product CRINA®, composed of a mixture of thymol, eugenol, vanillin, limonene, and guaiacol; the authors observed no effects on the microbial population, the production of volatile fatty acids, and methane production. According to the authors, the essential oils can be removed during normal rumen flow rates, and the dosage provided can be reduced to minimum concentrations that do not inhibit microorganisms. In addition, since essential oils have a low solubility, they can accumulate in the rumen environment and thus have a low effect.
Another possible explanation for the various results found in the literature is the adaptive capacity of rumen microorganisms to essential oils when supplied at low concen trations 10. The absence of the effect of essential oil supplementation was also verified by Simitzis et al. (2014), who evaluated the inclusion of oils in the diets of lambs and did not observe effects on feed intake, performance, and meat quality.
Several factors may affect the responses of supplementation with essential oils, namely the plant species used for the extraction of essential oils, the growth phase of the plant, the plant part (leaves, bark, flowers, roots, seeds), the growth environment, as well as soil composition, temperature, and light and water stress 10,12.
Moreno et al. (2021) reported that the inclusion of Allium sativa when associated in diets due to its organosulphurous compounds as allicin, which have antimicrobial activities act modulating the function of the rumen environment and favoring the synthesis of sulfur amino acids as methionine by the contribution of these compounds.
Rivaroli et al. (2016) evaluated the quality of the meat from animals consuming essential oils and observed that low doses reduce lipid oxidation and increase the shelf life of meat, when compared to diets without essential oils, due to the antioxidant effects of essential oils. In contrast, in high doses, essential oils act as pro-oxidants as they can increase the permeability of mitochondria, thereby damaging them and altering electron flow, which results in the production of more free radicals, such as reactive oxygen species 10.
Despite the demonstrated effects on the microbial population and, consequently, fermen tation and animal performance, these effects have only been studied for a limited number of oils, necessitating more studies evaluating the potential of different types of essential oils in animal feed. However, secondary compounds from plants, i.e., essential oils and tannins, can be used as natural products, without the issues mentioned for ionophores in ruminant production.
Tannins
Tannins are secondary compounds of plants characterized by phenolic rings and of variable molecular weight. They can form complexes with various substances due to the different forms of interactions such as covalent bonds, hydrogen bonds, or ionic bonds 61. These compounds are stored in the vacuoles of plant cells and have a defense function because of their antimicrobial and/or bacteriostatic characteristics, helping the plant reabsorb nutrients from injured cells or by inhibiting the action of predators 61.
Because of the antimicrobial and/or bacteriostatic properties, the use of tannin in the form of extracts or supplied through plants or plant parts containing tannin is widely used as an alternative method of rumen modulation, with the beneficial effects of methane miti gation, changing the biohydrogenation of polyunsaturated fatty acids 47,50,61.
Several studies have reported divergences regarding the tolerance of ruminants to the intake of diets containing tannin. In general, the tannin content varies between 4% and 5% of the dry matter of the diet. Supply occurs through plants containing tannin; if tannins are supplied via extracts, they are mainly in liquid or powder form. The chemical characteristics of the tannin and the protein, which are the salivary protein, or the protein from the other dietary components, may largely interact 50.
The use of tannin in ruminant diets a priori emerged as a way to increase the efficiency of the use of dietary nitrogen, mainly because through the tannin-protein bond, part of the protein is no longer degraded in the rumen and continues to the abomasum, where the reduction in pH promotes the tannin shutdown with the protein, leaving it available to be digested in the jejunum. In this way, the decreased degradation of proteins in the rumen can decrease the concentration of nitrogen in the rumen, in the form of ammonia and/or urea, thus reducing the excretion of urea and promoting nitrogen recycling and the use of essential amino acids in the diet 61. However, the presence of tannin in feed particles has negative impacts on gram-positive bacteria and Archaea, causing changes in the biohydro genation process 26,61.
Despite the positive effects mentioned above, some authors have reported that the addition of tannin caused a reduction in dry matter intake and in the digestibility of dry matter, protein, and neutral detergent fiber 27. Villalba et al. (2010) reported positive effects of the inclusion of tannins in ruminant diets and explained that because of the anti parasitic action of tannin, there was a greater integrity of the intestinal wall area, favoring the absorption of nutrients and, consequently, increasing the production of meat, milk, and wool. Tannin levels affect the use of the diet (intake and digestibility) and, consequently, the production of meat, milk, and wool. Regarding meat production, Vasta et al. (2009) reported an increase in the ruminal fluid of the concentration of conjugated linoleic acid and, conse quently, an increase in the concentrations of polyunsaturated fatty acids in the muscles of sheep. In their reviews, Ribeiro et al. (2011) and Morales & Ungerfeld (2015) stated that tannin more significantly affects the microorganisms that exert the last step of biohydroge nation, thus increasing the supply of 18: 1 fatty acid isomers to the small intestine.
Patra (2014) reported several studies in which the inclusion of tannin in the diets had no effects on the fatty acid profile of the milk of goats and sheep. However, Morales and Ungerfeld (2015) reported that the inclusion of tannin reduced the concentration of 18: 1 fatty acids and increased those of 18: 2 and 18: 3 fatty acids. In meat, tannin, when included in the lamb diet, increased the deposition of 18: 3, 18: 2, and 18: 1 and reduced the concen trations of saturated fatty acids 39,47.
When the tannin inclusion is associated with diets containing high levels of polyunsatu rated fatty acids, changes in the fatty acid profile of the product are promoted.
Chitosan
Chitin is the second most abundant organic compound on Earth and can be found in the cell walls of some fungi and algae as well as in the exoskeletons of some invertebrates such as crab and shrimp 31. Chitosan (polymer N-acetyl-D-glucosamine) is a natural biopolymer formed by the deacetylation of chitin in an alkaline medium 31 and mainly comprises 2-amino-2-deoxy-D-glycopyranose units. Chitin is composed of 2-acetamido-2-deoxy-D-gly copyranose units, both of which re linked by glycosidic bonds (Figure 5) 56.
Chitosan employment has increased exponentially in several areas, such as agriculture 53 or the food and pharmaceutical industry 11,15. However, its use as a feed additive in ruminant nutrition is largely neglected 15,18.
The effect of chitosan as an additive in ruminant nutrition was investigated by Goiri et al. (2010), using the rumen simulation technique (RUSITEC). The authors found that diets with chitosan do not alter the total production of volatile fatty acids but facilitate the production of propionate, resulting in a higher propionate: acetate ratio. Such modifications are relevant from an energy perspective and could improve feed efficiency in farm animals. Similar results were reported by Dias et al. (2017). An increase in the propionate: acetate ratio was also observed by Vendramini et al. (2016), who also found a reduction in methane production and a tendency to reduce fiber digestion, indicating a possible modification of the rumen microbial ecosystem, especially in fibrolytic bacteria.
The effects of chitosan supplementation on ruminal microorganisms were also investi gated by Goiri et al. (2010), who observed an inhibition of biohydrogenation fatty acids in the rumen, increasing the proportions of C18:1t11 (vaccenic acid) and conjugated linoleic acid and decreasing the proportions of saturated fatty acids. Alteration of the rumen microbiota can also have negative effects on dry matter digestibility, particularly in diets with a high amount of roughage, indicating that the effects of chitosan are greater on gram-positive bacteria 64.
Paiva et al. (2017) evaluated chitosan in the diet of cows and verified an increased milk yield as well as higher lactose and protein yields; however, the authors found no changes in the percentages of milk components. They attributed the high milk production to the greater supply of propionate, a gluconeogenic precursor. Garcia-Rodriguez et al. (2015) also reported higher concentrations of plasma glucose, suggesting a greater propionate production. Mingoti et al. (2016) reported that chitosan supplementation did not alter intake and milk yield and reported only slight changes in the milk fatty acid profile; these findings are different to those reported by Garcia-Rodriguez et al. (2015) and mentioned above.
Gandra et al. (2016) reported that chitosan supplementation reduces diet intake but increases dry matter digestion. Mingoti et al. (2016) found that diets with chitosan and soybean oil promoted an increase in milk yield and feed efficiency, reducing the profile of saturated fatty acids and increasing the levels of polyunsaturated fatty acids, mainly C18:2 cis-9,12, indicating changes in the biohydrogenation process.
Mingoti et al. (2016) explained that chitosan is capable of modifying rumen biohydro genation, but that the slight change in the profile of milk fatty acids found in this study is more related to the source of polyunsaturated fatty acids (whole soybean), which not being free, did not suffer the direct action of rumen biohydrogenation, because of this change in the profile was discreet considering the minor microbial action in the supply of fatty acids that reached the duodenum.
The mode of action of chitosan on microorganisms is not fully understood, and the theory of the intracellular extravasation mechanism is most accepted by the scientific community. In this theory, the positive charges of chitosan interact with the negative charges on the lipopolysaccharide surface of gram-negative bacteria and, similarly, with the peptidoglycan fraction of gram-positive bacteria 15. These interactions modify membrane permeability and promote the extravasation of intracellular components, causing bacterial death 15,21. However, these effects are less pronounced in gram-negative bacteria due to the presence of an outer membrane in these microorganisms; such a membrane is absent in gram-positive bacteria (Figure 6), making them more susceptible to the action of chitosan.

Figura 6: Vista esquemática de la pared celular de bacterias gramnegativas y grampositi vas, respectivamente (Adaptado de Nelson & Cox, 2014).
Conclusion
The additives presented in this review have different mechanisms of action in the rumen environment, either by changing the fermentation pattern and/or modifying the rumen microbiota, all of which are efficient in improving animal performance. There is an intrinsic relationship to the quality of meat and milk from ruminants, and these products are also related to the diet (concentrate: roughage rate, EE content, dietary fatty acid profile, dietary fiber quality).