Introduction
By assessing the presence of pollutants, land management strategies can reflect soil environmental quality. Unsustainable land management has led to critical levels of physical, chemical, biological, and ecological degradation of soil, at times reducing or eliminating the quality and primary functions of this resource 24,32. Pollution stands out among the forms of chemical degradation, considering the concentration of heavy metals in soil as an indicator of its chemical conditions 11,24,38. The term “heavy metal” refers to a range of metals and metalloids with an atomic density greater than 4 g cm -3; some with concentrations ranging from 0.1 to 18 mg kg -1 (7,25,30. Over 20 million hectares of soil worldwide are polluted with metallic and non-metallic elements, most notably As, Cd, Cr, Hg, Pb, Co, Cu, Ni, Zn, and Se 9,33. X-ray fluorescence (XRF) was among the approaches and devices used in this study to facilitate determinations. XRF spectrometry is an analytical method for determining the elemental composition of various materials. In soils, portable X-ray fluorescence (pXRF) spectrometry provides a wide range of pedagogical, environmental, and agronomic applications. Specifically, soil characterization by pXRF involves a comprehensive determination of its elemental composition (nutrients, trace elements, and rare-earth elements). XRF provides a rapid, cost-effective, and residue-free assessment of soil properties that allows for assessment of a more significant number of samples and a more in-depth characterization for different purposes 22,33,36. A number of studies in the literature have reported on the determination of Ti, V, Cr, Mn, Ni, Cu, Zn, Zr, Sr, Rb, Pb, and Th contents using this technique 33,35,36,41.
In Mexico, the toxicity and abundance of some of these elements, primarily due to increasing mining activities, have turned heavy metal pollution into a growing concern, particularly in the states of Zacatecas, Queretaro, Hidalgo, and San Luis Potosi 15. Sierra de Alvarez is critical to San Luis Potosi due to its diverse climate and vast variety of vegetation in its temperate, arid, mountainous, and sub-humid landscapes. Predominant soil types include Eutric Lithosol, Haplic Luvisol, Rendzina, and Luvic Phaeozem. The intrinsic effects of agriculture, livestock production, and mining activities practiced 20 there have had a dramatic impact on the state of the environment and are reflected in the decline in ecosystem services 29. Given the human influence on land use, this area has been of interest for soil restoration plans since 2004 under the Mexican Environmental Compensation Program (CONAFOR) 14. Our study was conducted in the communal lands of Monte Caldera, within the municipality of Cerro de San Pedro, San Luis Potosi, to assess presence of metallic and non-metallic elements as indicators of the environmental impact of land uses and evaluate remediation strategies for polluted soil. Approaches to the restoration of soil quality include conservation agriculture, integrated nutrients, continuous management of vegetation cover, cover crops, diversified land use, controlled grazing, and optimal seeding rates, among others 24. To assess the impact on soil quality and determine the degree of restoration in the area under study, we examined the characteristics of land use, i.e. livestock production, restoration practices (sites with mechanical practices, specifically the formation of terraced reforestation with Pinus greggii, and livestock exclusion), and overgrazed land without management or conservation practices.
Materials and methods
The research was conducted in the communal land of Monte Caldera, which is part of the Cerro de San Pedro municipality in the state of San Luis Potosi, Mexico. Monte Caldera is located at 100°44’4” west longitude; 22°12’31” north latitude; at 2080 meters altitude 17,21.
Biotic Characterization
The predominant climate in Monte Caldera is temperate semi-arid. Average annual temperature in the study area is 16.8 °C with an annual rainfall of 304 mm. The municipality of Cerro de San Pedro is located in El Salado hydrological region (HR 37), which essentially consists of a series of small endorheic basins (Cuenca San Jose, Los Pilares, and others). The region is bordered by a massif of sedimentary rocks with primarily pine-oak vegetation, induced pasture, and grassland use in the upper part 21. The soil types present are Lithosol, Chromic Luvisol, Calcareous Regosol, and Mollic Planosol 26.
Site Selection
The assessment was conducted consecutively in July 2018 with a completely random sampling of three land uses: 1) Land use with managed livestock production; 2) Land use with restoration practices, i.e., land implementing soil rehabilitation measures established in 2004 under CONAFOR, with reforestation with Pinus greggii and fencing 14, and 3) Land without livestock management or conservation activities, corresponding to continuous grazing. Each land use was georeferenced in the field with a global positioning system, considering a total area of 1,124 ha ( Figure 1).
Soil physicochemical characteristics
Six sampling points were selected for all three land-use practices studied, 15 to 20 meters apart from one another and completely randomly collected. Six samples and three replicates were collected for each land use at each sampling point using two depth criteria: 0-10 cm and 10-20 cm, reaching 36 samples in total. Soil properties were analyzed using the techniques established in the Mexican Official Standard NOM-021-SEMARNAT-2000 13,18. Analyses of pH, texture, soil organic matter (SOM), electrical conductivity (EC), and cation exchange capacity (CEC) were performed in triplicate for each sampling site. Total concentrations of Zr, Sr, U, Th, Pb, As, Rb, Cr, V, Ti, Zn, and Cu were calculated using X-ray fluorescence with a Thermo Scientific Niton TM FXL instrument (Thermo Fisher Scientific, Waltham, MA., USA). The results for each sample were based on the average of three replicates with a 60-second analysis time. An internal calibration of the instrument was performed before analyzing the samples, using standard reference soil material from the National Institute of Standards and Technology (NIST). The procedure was performed in accordance with manufacturer’s instructions and recommendations of the U.S. Environmental Protection Agency (EPA) SW-846 Test Method 6200 39,40,41.
Statistical analysis
Minitab 16 Statistical Software (Minitab Inc., State College, PA., USA) was used for data analysis. A model was designed considering the effect of land use factors and soil depth on 12 metallic and non-metallic elements, determining the SOM, EC, and CEC soil indicators with Pearson’s correlation coefficient, ANOVA (Tukey’s test, p≤0.05) and a Principal Component Analysis (PCA) for mean comparisons. To minimize error, readings of the soil samples were made in triplicate.
Results and discussion
The average SOM behavior of all samples assessed was 4.14%, EC was 0.33 mS cm -1, and CEC was 15.86 Cmol kg -¹. The pH ranged from 6.5 to 7. The soil textures found ranged from loam (Livestock management) to silt and clay loam (Restored soil), and to loam and clay loam (Land without management or conservation). Overall mean values for the 12 elements were as follows: Ti (3,839.1 mg kg -1) > Zr (518.2 mg kg -1) > Rb (111.19 mg kg -1) > V (79.83 mg kg -1) > Sr (72.33 mg kg -1) > Zn (60.84 mg kg -1) > Cr (42.60 mg kg -1) > Pb (19.64 mg kg -1) Cu > (17.13 mg kg -1) > Th (11.51 mg kg -1) > U (6.94 mg kg -1) > As (6.13 mg kg -1). Table 1 (page 57) illustrates the mean soil concentrations of these elements. Cr, U, and Th values exceeding the technical reference values for concentrations considered phytotoxic are marked as relevant 1,19,31.
Table 1: Mean concentrations of metallic and non-metallic elements in soils and reference values (n=36). Tabla 1: Relación de concentraciones medias de elementos metálicos y no metálicos en suelos y niveles de referencia (n=36).
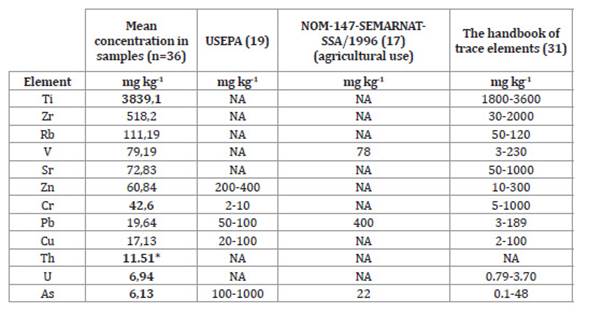
Note: Mean metal concentrations refer to the total samples from the different land uses evaluated. NA=Data is not available in the technical reference. * Mean soil concentration of Th is 6 mg kg -1 (1.
Nota. Los niveles de concentraciones medias de metales refieren el total de muestras de los diferentes usos de suelo evaluados. NA. Dato no disponible en la referencia técnica. La concentración media de Th en suelo es de 6 mg kg -1(1.
Several works in the literature have reported varying concentrations of U and Th in soils in countries such as the United States, Canada, Germany, Jamaica, Cuba, India, and Egypt 17. Considering the values presented in Table 1 (page 57), it is worth noting that the Th contents measured in our study were within the means reported for soils in the United States (2.2-21 mg kg -1), Canada (4.2-14.1 mg kg -1), Germany (0.4-15 mg kg -1), Jamaica (0.9-25 mg kg -1), and Cuba (5-12.3 mg kg -1). The mean values for U in soil obtained in this study were consistent with those obtained for soil from the United States (0.3-10.7 mg kg -1), Germany (0.42-11 mg kg -1), and Jamaica (0.7-14 mg kg -1). The presence of any element at higher or lower concentrations in a given soil is strictly contingent on its mineral composition, nature of the original sediments, and depositional environment 18. There were 113 interactions, 20 of which were significant according to Pearson’s correlation coefficient (p≤0.05, Table 2, page 57).
Table 2: Relationship between metallic and non-metallic elements and soil physicochemical characteristics. Tabla 2: Relación entre elementos metálicos y no metálicos y propiedades fisicoquímicas del suelo.

* Indicates statistically significant relationships (p≤0.05).
Note: Values with ** refer to highly significant r values, those with * to significant r values.
* Indica relaciones estadísticamente significativas (p≤0,05).
Nota: Valores con ** se refieren a r altamente significativa, valores con * se refiere a r significativa.
Clay, calcium carbonate, SOM, and pH are among the constituents or properties that contribute to the presence of these elements in soil 39. Heavy metals exist in most soils as carbonates, sulfides, oxides, or salts, and their concentrations may vary from soil to soil 35. In our study, however, significant correlations (p≤0.05) with positive or negative signs were observed for the SOM, EC, and CEC soil parameters, with the highest values being those recorded between SOM-Zn (r=0.41) and SOM-Ti (r=-0.37). In turn, pH and organic carbon had the most significant influence on the solubility of metals 34. In other studies, soil chemical properties such as pH and EC have been found to vary depending on practices such as forest use and conservation. Their effect can be explained by an increase in soil cover, which decreases with the intensity of agricultural use 10. In addition, positive relationships have been observed among elements such as Fe, Cr, Mn, Ni, As, Cu, and Zn, As, and Cr and between these elements and soil constituents or properties such as clay, calcium carbonate, organic matter and pH 39.
Tukey’s test (p≤0.05) showed significant differences in Th, Pb, Rb, and Cu among the means for the different land uses analyzed by our study ( Figure 2).

Different letters indicate significant differences.
Medias que no comparten una letra son significativamente diferentes.
Figure 2: Mean values among significant element concentrations in relation to land use (p≤0.05, n=36). Figura 2: Medias de los elementos con concentraciones significativas según usos de suelo (p≤0,05, n=36).
The samples corresponding to livestock management had the most significant mean values for Th (12.97 mg kg -1), Pb (22.0 mg kg -1), Rb (119.12 mg kg -1), and Cu (19.21 mg kg -1). In like manner, SOM concentrations were significant at a rate of 4.74% in restored soil, 3.97% in livestock management, and 3.31% for areas without management or conservation. Specifically, SOM (humic and fulvic acids) in soil is a component with a high sorption capacity for heavy metals, impacting their immobilization 23,28.
A difference of 4 mg kg -1 in copper (Cu) content was observed between land use with livestock management and land use with restored soils. Cu is a metal that occurs naturally in rocks, soil, water, and air. Agricultural activities and wastewater discharge into rivers and lakes also contribute to its release 3. In the Handbook of Trace Elements, Pais and Jones (1997) indicate that the total content of Cu in soil ranges from 2 to 100 mg kg -1; Cu content exceeding phytotoxic levels ranges from 20 to 100 mg kg -1 (14,19. The Canadian Soil Quality Guidelines (CSQG) for the Protection of Environmental and Human Health assign a soil quality guideline of 63 mg kg -1 as necessary to protect the environment 12. As for thorium (Th), small amounts of this element occur naturally in the environment in rocks, soil, water, plants and animals; soil contains an average of 6 mg kg -1 (1. Lead (Pb) levels detected by our analysis were below the limit for agricultural use, which is 400 mg kg -1 according to the Mexican Official Standard NOM-147-SEMARNAT/SSA1-2004 (2007). Its total concentration in the soil ranges from 3 to 189 mg kg -1 (31; therefore, the value found for this element does not exceed technical reference values for concentrations considered phytotoxic, which are 50 to 100 mg kg -1. In terms of Rubidium (Rb), the Handbook of Trace Elements reports that the total content of this element in soil ranges between 50 and 120 mg kg -1 (31. Finally, it should be noted that some metals such as Cu, Pb, Zn, and As are absorbed by grassland. It has been found that cattle can involuntarily ingest from 1% to nearly 18% of soil within dry matter, which can vary depending on season and farm management. Heavy metals may be found in their feces, contributing to their spread 37.
A significant effect of the soil depth factor was found in relation to U, Pb, As, and Zn concentrations ( Figure 3).
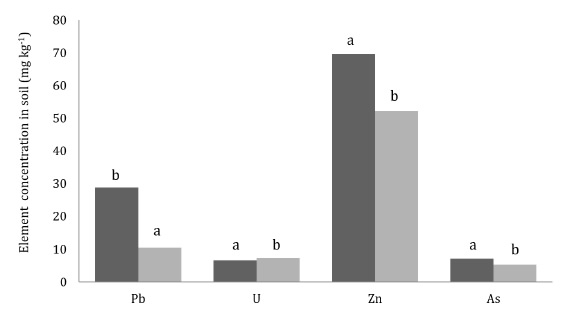
Different letters indicate significant differences.
Medias que no comparten una letra son significativamente diferentes.
Figure 3: Mean values among significant element concentrations in relation to land use (p≤0.05, n=36). Figura 3: Medias de los elementos con concentraciones significativas según usos de suelo (p≤0,05, n=36).
Pb, As, and Zn values were notably higher at a depth of 0-10 cm, and only U values were higher at a depth of 10-20 cm. According to the Handbook of Trace Elements, the total amount of uranium in the soil ranges from 0.10 to 11.2 mg kg -1 (30. Uranium is released into the soil when rocks are eroded by wind or water 2,6. As an element widely distributed in soils around the world, total soil concentrations of Arsenic (As) range from 0.1 to 48 mg kg -1 (5,13,27. The concentration found in this study is below the value of 12 mg kg -1 established by the CSQG, therefore it does not affect soil quality 12. The total concentration of Zinc (Zn) in soil ranges from 10 to 300 mg kg -1. Natural processes release a small amount of Zn, whereas human activities such as mining and steel production account for its total amount 4. Significant concentrations of Zn were observed in our study, ranging from 52.13 to 69.54 mg kg -1, and were higher in the soil samples collected at 10 cm depth, with a difference of 17.41 mg kg -1 between the two studied depths. According to EPA guidelines (1992), these levels of Zn do not exceed reference values for concentrations considered phytotoxic.
It is essential to relate the presence of SOM to these results, since concentrations obtained considering the depth factor were significant. Compared to the value established in NOM-021-SEMARNAT-2000 (2002), SOM concentrations were high, ranging from 3.6 to 6.0%, for all land uses and soil depths applied in this study. Soil samples had an average of 4.5% SOM content at 0-10 cm, and 3.73% at 10-20 cm, representing a difference of 0.82% between both soil depths. In terms of land use, SOM concentration was 4.74% in restored land, representing the highest value, 1.03% more than that for managed livestock production activities, which was 3.71%. A decrease in SOM with agricultural and livestock production practices may be due to changes in stocking capacity, removal of crop residues, and more rapid decomposition, oxidation, and soil erosion processes. In contrast, an increase in organic matter has been observed in areas where soil conservation practices are developed 10. Organic carbon and CEC are the two main factors that suppress the effect of toxicity on soil respiration in heavy metal-polluted soils, with Zn, Cu, and Pb being the most prominent elements 33. Humic and fulvic acids derived from organic matter are beneficial because of their high absorption capacity for various contaminants, including heavy metals, which can result in their immobilization 22.
According to the guidelines set by NOM-147-SEMARNAT/SSA1-2004 (2007), the Pb and As contents we found do not exceed the limits for agricultural use, which are 400 mg kg -1 and 22 mg kg -1, respectively. The presence of these elements may be influenced by the origin and composition of soil, as well as by the impact of human activities such as livestock production and agricultural uses and external environmental factors.
The highest and lowest concentrations considering land use (for Th, Pb, Rb, and Cu) and soil depth (for Pb, U, Zn, and As) may be related to the natural composition and mineral structure of soil and human activities. In this study, agricultural and livestock production activities affected metal concentrations; however, except for Pb concentrations, our findings were influenced by both land use and soil depth. In soils where restoration and reforestation activities occurred, in addition to a higher concentration of organic matter, there were lower concentrations of these elements. Higher organic matter content implies greater nutrient availability from mineralization of organic compounds, allowing for a higher rate of microbial development, and consequently lower metal stress in polluted soils 34.
Two data groups were identified with component analysis, which may explain the variation in the significant presence of metallic and non-metallic elements based on the land uses and soil depths assessed ( Table 2, page 57 and Figure 4, page 61).
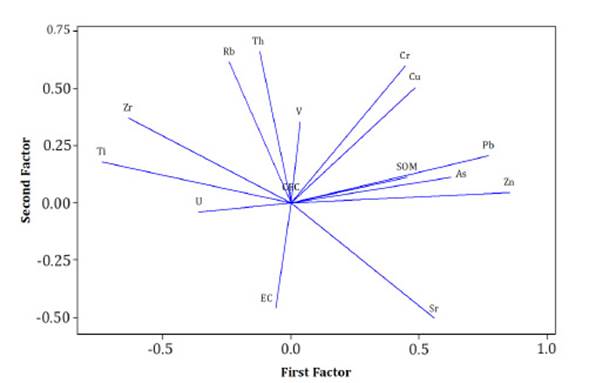
Figure 4: Loading plot of metallic elements, non-metallic elements and soil properties derived from the factor analysis. Figura 4: Diagrama carga de los elementos metálicos, no metálicos y propiedades del suelo derivado del análisis de factores.
This variation may be associated with the physicochemical dynamics, composition, and loam-clay texture of the soil in the different land uses, with Pb, Cu, Th, Zn, As and SOM being strongly related to this variation. This may have an environmental impact on homogeneity, primarily due to land use with livestock production and agricultural activities, soil restoration practices, and soil conservation. The percent SOM was significant in land with restoration practices such as reforestation, rehabilitation of gullies, and regeneration of vegetation, among others. This could be an essential factor in the oxidation, adsorption, or retention of metals in soil. Assefa et al. (2020) indicate that assessment of the physicochemical properties of soil associated with overgrazing, absence or limitation of fallow periods, steep slopes, and land-use management practices, is related to an understanding of soil status and quality 10. In the results presented in Table 3 (page 61) and Figure 4 (page 61), Factor 1 illustrates the data relationship explaining 38% of the variance in our study, related to the soil samples with the highest concentration of Pb, Zn, As, and Sr (positive charges) and of Ti and Zr (negative charges). Factor 2 is associated with Th, Rb, and Cr (positive charges) and with Sr and EC (negative charges), explaining 22% of the accumulated variation.
Table 3: Variable loading coefficient (vectors) of the first three factors using 15 variables related to land use (livestock management, restoration, and land use without management or conservation). Tabla 3: Coeficiente de carga de variables (Vectores), de los tres primeros factores utilizando 15 variables de acuerdo con los usos de suelo (manejo de ganado, restaurado y sin manejo o conservación).

Factor loads in bold (> 0.70) are considered highly weighted. SOM=Soil Organic Matter, EC= Electrical Conductivity, and CEC=Cation Exchange Capacity.
Las cargas de factores en negrita (>0,70) se consideran altamente ponderadas. MOS (Materia Orgánica del Suelo), CE (Conductividad eléctrica), CIC (Capacidad de Intercambio Catiónico).
Factor 3 explains 19% of such variation and was more closely related to CEC, V, and Ti. Regarding the analysis of factors, the three determining factors account for 80% of the variation, indicating a relationship among soil properties (EC, CEC, and SOM), land use and soil depth ( Table 2, page 57 and Figure 4, page 61). The similarity analysis found relationships between metallic and non-metallic elements and soil parameters of 58.64% to 88.98%, with a particularly notable relationship between Zr-Ti and Pb-Zn (positive charges). The lowest percentage, namely 58.64%, was observed between EC and the rest of the elements and properties, 64.92% for CEC and V-Zr-Ti; and the closest interactions were those between SOM with Pb-Zn-As and Cr-Cu ( Figure 5, page 62).
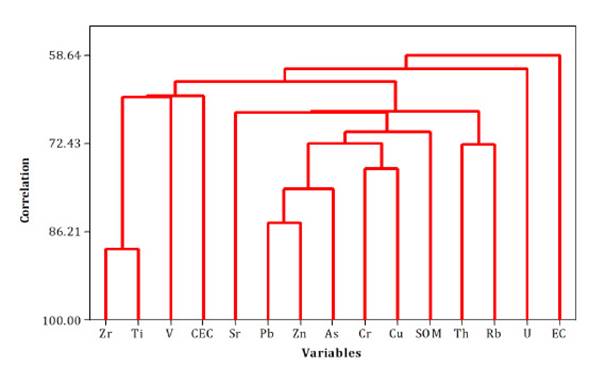
Figure 5: Dendrogram derived from the correlation coefficient between metallic and non-metallic elements and soil properties. Figura 5: Dendrograma derivado del coeficiente de correlación entre elementos metálicos y no metálicos y propiedades del suelo.
This result is associated with the correlation analysis presented in Table 1 (page 57). Our results are consistent with similar PCA results and correlations, indicating that elements such as As, Cr, Fe, Mn, and Ni could be of lithogenic origin, while Cu, Pb, and Zn may be due to human influence 8,39.
Conclusion
The land use factor related to livestock production, restoration and conservation confirmed the presence of 12 metallic and non-metallic elements, which may be attributed to the natural origin of soil and the dynamics of human activities in the region studied. The SOM value was classified as high in accordance with Mexican regulations for soils, principally due to soil remediation practices that have had a positive environmental impact on modulating heavy metals. Notably, As, Pb, and Zn levels did not exceed the limits set by Mexican regulations and international references in soils with these land uses. Based on technical considerations, the total concentrations of Th, Pb, Rb, Cu, Sr, As, and Zn in soil were within permissible limits. However, Ti, Cr, Th, U and As exceeded the technical references for soil concentrations considered phytotoxic. Livestock production practices had a notable impact on the concentrations of elements such as Th, Pb, Rb, and Cu. Land use activities with remediation practices and without management contribute to the low availability of metallic and non-metallic elements in the studied region. These findings underscore the need to continue technical research and monitor environmental impact as related to other natural resources and land uses.