Introduction
Genetic improvement via the induction of mutation has been applied to various plant species that have nutritional importance or ornamental interest 27,42. To induce mutations, physical and chemical agents, or a combination of both, have been used. Determining the mean lethal dose (LD50) is essential 54. The LD50 value represents the amount of radiation or chemical agent absorbed with which 50% of the exposed population survives or that causes 50% growth reduction 7. Mutagens must be used in accordance with the aforementioned doses, since a greater 5 spectrum of mutations is generated; moreover, lower doses (less than 300 Gy) allow recovery of an adequate number of useful mutants compared to higher doses 29.
Compared to other types of radiation, the induction of mutations by gamma radiation has prevailed due to its wide availability, versatility and greater effectiveness at generating a wide range of mutations 9. The penetration of gamma rays into plant tissues is strong and uniform, which results in its high potential for plant breeding 28,33. Exposure to ionizing radiation activates a series of steps between the initial absorption of energy and the final biological injury. The main and most important direct targets of radiation are water molecules, which lead to the generation of chain reactions that produce reactive oxygen species (ROS). These radicals are toxic at high levels and can react rapidly with various types of macromolecules, including lipids, proteins and, in particular, DNA, leading to cell damage and cell death 14. Data from the International Atomic Energy Agency 26 through 2017 revealed that 3,251 registered plant varieties were generated from induced mutations, of which 49.4% were obtained by gamma radiation.
The Selliera genus consists of three perennial rhizomatous herbaceous species that are currently recognized as a single polymorphic group 44. The species Selliera radicans Cav. has a rosette-type growth habit with whole, green fleshy leaves 22. The species is used as an ornamental plant for low-maintenance coverings, and due to its ability to be propagated by stolons, it is used in the creation of green roofs 51. This species can tolerate strongly saline soils that have an electrical conductivity > 20 dS m-1 and a pH ranging from 5 to 10 3. Due to the scarcity of good-quality water resources required by ornamental species, especially those used for forage, the use of this type of species represents a great opportunity 12. The Universidad de Talca (UTalca) and the Pontificia Universidad Católica de Valparaíso (PUCV) currently have a collection consisting of 11 accessions from different areas of Chile; most of these accessions were described by Meza et al. (2015).
The aim of this study was to determine the effects of different doses of gamma radiation on the seed germination and development of seedlings (M1) and the LD50 of two accessions of Selliera radicans for use in the induction of mutations.
Materials and methods
Plant material
Fresh seeds were extracted from the dried mature fruits of individual plants that were maintained as an in vivo clonal collection of plants, were collected from different zones in Chile and had been propagated for three generations. The selected accessions for this study were Vichuquén and La Serena.
Irradiation tests
Radiation was carried out on the premises of the Comisión Chilena de Energía Nuclear (CCHEN), La Reina, Santiago, Metropolitan Region, Chile. A 60Co Gammacell 220R irradiator was used. The irradiation geometry was previously determined, and via Fricke dosimetry, the necessary time of exposure to apply the required doses was also determined. To determine the LD50, seven doses were applied: 100, 200, 300, 400, 500, 600 and 700 Gy. A nonirradiated seed control group was also included. For each dose, 100 seeds were used. The seeds were sown one day after irradiation.
Sowing and germination conditions
The seeds were placed in Petri (10 cm) dishes on filter paper that was moistened with disinfectant solution (1 g L-1 captan); four replications of 25 seeds were used for each dose. The seeds were stratified for two weeks at 8°C in darkness according to Schiappacasse et al. (2017). Afterwards, the Petri dishes were transferred to germination conditions, which were 20°C (± 2°C) and artificial lighting for 24 h per day at 80 μmol m-2 s-1. Germination was recorded weekly for 12 weeks. Seeds that produced a radicle equal to or greater than 2 mm were considered germinated 50.
Seedling growth and development conditions
Seedling size was determined by measuring the total length and shoot and root length from the apex of the cotyledons to the distal root end via images of the seedlings. Subsequently, all the seedlings were planted in trays filled with peat and perlite at a ratio of 1:1 (v/v); the substrate was amended with a Multicote© granular fertilizer (5 g L-1). The seedlings were then kept under greenhouse conditions (21.2°C and 58.1% relative humidity [RH]). Two hundred days after germination, the final length of the seedlings was measured by digital image analysis using ImageJ© software, and the percent survival, in accordance with dose, was determined.
Statistical analysis
The tests were conducted in accordance with a completely randomized model; for both accessions, each dose was applied to a total of 100 seeds (4 replications with 25 seeds for each replication in a Petri dish). Germination curves were adjusted for each combination of dose and accession separately. The germination rate at time t was modeled using a log-logistic model with three parameters via the drc package in R software version 3.4.3 48.
The parameter d indicates the maximum proportion of germination, e is the median time of germination (when F (t) = 0.50), and b is proportional to the slope of F (t) when t = e. The estimation and verification procedures of the models are based on the use of time data for the event of interest, in this case, germination, as described by Ritz et al. (2015).
To determine the LD50, a dispersion graph was constructed that included the cumulative germination at the end of the test (y-axis) in response to the applied radiation dose (x-axis). A linear relationship between both variables was calculated, and the LD50 value was then determined. The results of the survival lengths and percentages were processed with Minitab 17 statistical software. The seedling length values were transformed to 1/x values 41. The data were examined via analysis of variance (ANOVA), and means were compared by Tukey’s test. For all the analyses, a significance level of 0.05 was used.
Results
The results of the model of each accession are shown in Table 1 and Table 2.
Table 1: Tabla 1: Estimates of the parameters (e, d y -b) and standard errors (SEs) of the log-logistic model as a function of the dose of gamma radiation for accession Vichuquén of Selliera radicans1. Estimaciones de parámetros (e, d y -b) y error estándar (ES) del modelo log-logistic en función de las dosis de radiación gama para la accesión Vichuquén de Selliera radicans modelo 1.
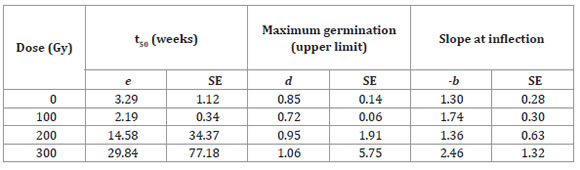
Table 2: Tabla 2: Estimates of the parameters (e, d y -b) and standard errors (SEs) of the log-logistic model as a function of the dose of gamma radiation for accession La Serena of Selliera radicans2. Estimaciones de parámetros (e, d y -b) y error estándar (ES) del modelo log-logistic función de las dosis de radiación gama para la accesión La Serena de Selliera radicans modelo 2.
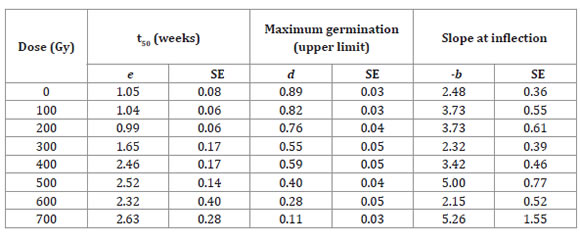
For the Vichuquén accession, it was only possible to model the 300 Gy dose due to the large number of seeds that did not germinate at higher doses; the lower radiation doses had a stimulatory effect. For the La Serena accession model (Table 2), parameter d (maximum germination ratio) tended to decrease as the dose increased, and parameter e (mean germination time relative to parameter d) increased as the dose increased.
Under the control conditions, the germination percentage was 65% for the Vichuquén accession and 89% for the La Serena accession. The Vichuquén accession reached its maximal germination during the eighth week (at the 100 Gy dose); on the other hand, the La Serena accession reached its maximal germination during the fourth week (at 0 Gy). The increase in dose had a negative effect on germination. When comparing both accessions, Vichuquén was more radiosensitive, since doses greater than 200 Gy were drastically harmful, and doses greater than 300 Gy resulted in no survival. For this accession, there was a stimulatory effect on germination at a dose of 100 Gy; these results were similar to those of the control (Table 1).
Compared with the Vichuquén accession, the La Serena accession was more radiotolerant, having higher values in the germination curves. In agreement with the results of the other accession, the most harmful dose for La Serena was 700 Gy, but in this case, total lethality was not reached at any dose (Table 2, page 39).
The differences in the germination curves of both accessions were converted into calculated LD50 values, as shown in Figure 1a.
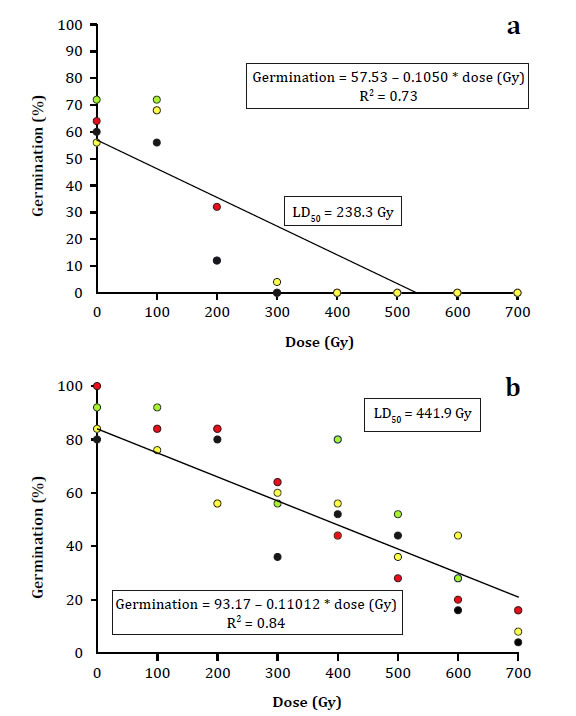
The percentage of germination used corresponding to week 8 (4 replicas of 25 seeds each).The boxes within the graphs show the equation of the trend lines and the LD50 values. Figure a corresponds to accession Vichuquén; Figure b corresponds to accession La Serena.
El porcentaje de germinación utilizado corresponde a la semana 8 (4 réplicas de 25 semillas cada una). Los recuadros dentro del gráfico muestran la ecuación calculada y el valor estimado de LD50. La Figura a corresponde a la accesión Vichuquén y la Figura b a la accesión La Serena.
Figure 1: Figura 1: Radiosensitivity curve for Selliera radicans accessions Vichuquén and La Serena. Curva de radiosensibilidad para las accesiones Vichuquén y La Serena de Selliera radicans.
The greater radiosensitivity of the Vichuquén accession was reflected in its LD50 value of 238.3 Gy, which was lower than that calculated for the La Serena accession (441.9 Gy).
Although germination was observed for both accessions in response to different doses, survival and growth occurred only with the 0 and 100 Gy doses, and dose significantly affected the percentage of seedlings formed (Figure 2, page 41).
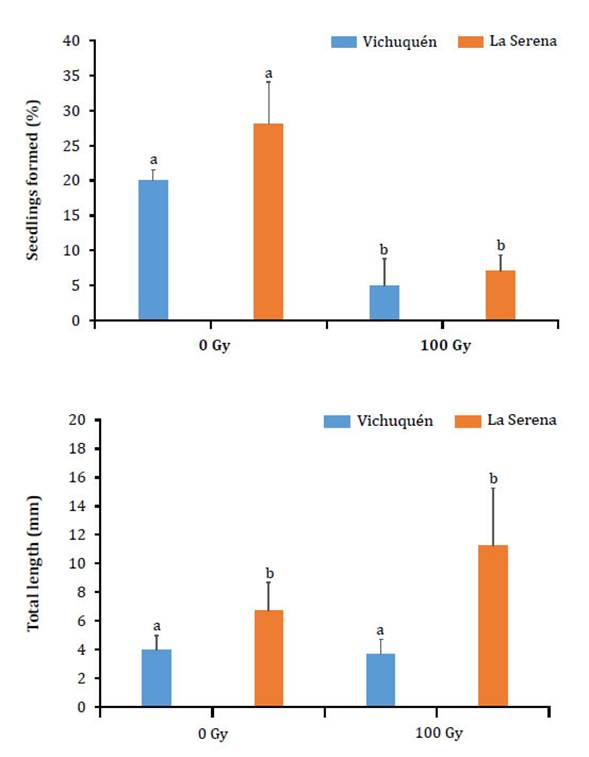
The bars indicate the standard deviation, and different letters denote significant differences (Tukey test, α = 0.05%).
Las barras indican la desviación estándar y las letras diferentes indican diferencias significativas (Test de Tukey, α = 0,05%).
Figure 2: Figura 2: Percentage and length of seedlings formed by the Selliera radicans accessions Vichuquén and La Serena. The percentage of seedlings was estimated in terms of the total seeds of each replication (25 seeds) at 12 weeks after sowing. Porcentaje y longitud de plántulas formadas en las accesiones Vichuquén y la Serena de Selliera radicans. El porcentaje de plántulas fue estimado con base en el total de semillas de cada réplica (25 semillas) a las 12 semanas posteriores a la siembra.
At the end of 12 weeks, the length of the control seedlings of the Vichuquén accession did not surpass 4 mm, and those of La Serena were significantly longer. Compared with the control dose, the 100 Gy dose had a stimulatory effect that resulted in longer La Serena seedlings. No mutations with obvious morphological effects, such as changes in leaf color or deformities in the formed seedlings, were observed in the M1 seeds.
At 200 days after transplanting, data are only available for the Vichuquén accession since the seedlings from La Serena did not survive the radiation stress. The survival percentage according to dose reached 55% for the control and 60% for 100 Gy with respect to the total number of transplanted seedlings, with no significant differences. Regarding the values achieved in terms of leaf and root length, there were no significant differences between the control dose and the 100 Gy dose. The total length (foliar and root lengths) of the seedlings after 200 days showed an approximately 30-fold increase with respect to the initial seedling size (Figure 3, page 42).
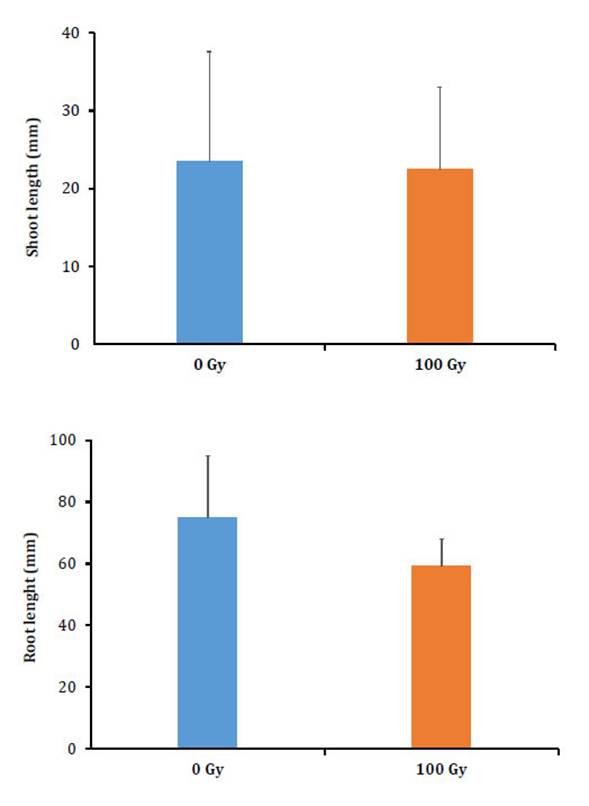
The measurements correspond to all the seedlings formed at 200 days after transplanting. The bars indicate the standard deviation.
Las medidas corresponden a todas las plántulas formadas 200 días después del trasplante. Las barras indican la desviación estándar.
Figure 3: Figura 3: Foliar and root length for the Selliera radicans accession Vichuquén. Longitud foliar y radicular de Selliera radicans accesión Vichuquén.
Discussion
At higher doses of gamma radiation, a loss of viability was observed in the seeds of Selliera radicans, especially for the Vichuquén accession, which resulted in a decreased germination percentage (Tables 1 and 2, page 39). This phenomenon may be related to the frequency of chromosomal damage, as an increased dose may be responsible for a lower germination potential and subsequent reduced growth 31,37,39. Gamma radiation-induced DNA damage results in the activation of different mechanisms to identify both the type and level of damage caused and drive both the repair and separation of damaged bases 17,18. Depending on the radiosensitivity of each genotype, the levels of damage to the cells and cell protection involve a cascade of events that imply the activity of a series of enzymes and genes 25.
Ionizing radiation triggers a series of enzymatic defense systems (peroxidases and superoxide dismutases) and nonenzymatic systems in plants; these systems are involved in the compensatory mechanisms of free radical inhibition formed by oxidative stress after exposure to gamma radiation 2,10,21.
Oxidative stress at certain intensities can be repaired at the cellular level; however, gamma radiation affects the rate of germination (Tables 1 and 2, page 39) and causes less growth as the dose increases. This pattern has also been reported in Chenopodium quinoa19, Zea mays36 and Solanum melongena8. The 100 Gy dose stimulated the germination of the Vichuquén accession (Table 1, page 39). Similar results in response to 100 Gy doses have been reported for Terminalia arjuna1 and Moluccella laevis40.
The stimulating effects of radiation on germination are still being studied. Some theories point to stimulating effects on enzymatic activity and on the synthesis of nucleic acids or the activation of protein synthesis; these effects occur during the initial stage of germination after irradiation of the seed, accelerating cell division and directly or indirectly stimulating auxin-response genes 7,35.
Because the ability to repair damaged DNA has a strong genetic component, the studied accessions have specific radio sensitivities in terms of germination (Figure 1, page 40). Differences in LD50 values have been recorded for other species at the variety or accession level 4,30,40,52. In Vigna unguiculata, the LD50 value varied between 165 and 689 Gy depending on the genotype used 23.
Compared to that at the 100 Gy dose, the formation of seedlings in response to the 0 Gy dose was evidently greater (Figure 2, page 41). It has been observed that at an early stage, growth is interrupted since the seedlings that form cannot survive after a certain period, which is probably due to DNA breakage and the inability to repair the damage 11,20,53. The prolongation of the growth interruption period can vary from a few minutes to several days, mainly according to the irradiation dose and the intrinsic radiosensitivity of the organism 34.
The imbalance in the development from the emergence of the radicle to the formation of a seedling may be related to an interruption of the cell cycle in G2/M phase during somatic cell division and/or to damage within the genome 45. In addition, imbibition is not affected by mutagenesis; this process has a relatively more pronounced effect on the subsequent development of the primary structures of seedlings, which can guarantee their survival 16. Interruption of the growth of the radicle and the zone of active cell division frequently 15 results in abnormal cells that have chromosomal aberrations 6.
The La Serena seedlings that survived the 100 Gy dose were longer than the control seedlings. A similar phenomenon was reported in response to gamma irradiation of Vigna unguiculata seeds at this same dose; this irradiation increased the vigor of M1 seedlings in terms of foliar area and plant height at six weeks 43.
The ability of radiation to increase secondary metabolites may be because gamma radiation is a potent inducer of metabolites that have known activities against a broad spectrum of plant stresses, particularly oxidative stresses 47.
Considering the doses that allowed the formation of true viable seedlings, future applications of gamma radiation in this species should focus on doses lower than 200 Gy. In general, most mutated genes are recessive; it has been reported that the frequency of recessive mutations ranges from 90 to 100% and that for dominant mutations ranges from 0 to 6% 16. From the M2 generation onwards, the resultant individuals are genetically diverse due to phenotypic segregation, which allows extensive selection from generations M2 to M5 to be carried out 24.
One of the most crucial requirements when inducing mutations is the selection of effective doses of mutagenic agents applied to the parental materials. This fact indicates why the significant differences between the LD50 values established in this study represent an important factor for subsequent irradiations, as the range of doses that can be used is limited 32. Selliera radicans was shown to be very sensitive to gamma radiation; in this respect, there may be a relationship between genome size and radiation sensitivity 13.
The ornamental potential of this species could be improved by gamma radiation, as many attractive characteristics that can be modified, such as growth habit and flower color and shape, are easily observable if they are present in plants generated after mutagenic treatment 46.
Conclusions
For the Vichuquén accession, the LD50 value for M1 seed germination was 238.3 Gy; for La Serena, it was 441.9 Gy. However, Selliera radicans was highly sensitive to gamma radiation, so seedling formation occurred only at a dose of 100 Gy because the seedlings of La Serena did not survive after 200 days. At low doses, there was a stimulatory effect that could be studied in future research to accelerate the germination process. This beneficial effect did not translate into an increase in the foliar or root length of the seedlings formed at the dose of 100 Gy for the Vichuquén accession.